Nano-magnesium phosphate hydrogels: efficiency of an injectable and biodegradable gel formulation towards bone regeneration
The use of autografts is considered as gold standard for the treatment of bone tissue defects caused by tumor, disease or trauma. The second surgery for autologous bone harvesting is often linked to donor site morbidity, pain, blood loss and infections (1). To circumvent these issues, synthetic bone grafts made of either organic biopolymers such as poly lactic-co-glycolic acid (PLGA) or collagen (2) and inorganic replacement materials like calcium orthophosphate (CaP) bioceramics are commercially available and frequently used in the clinic (3). The variety of such materials in terms of composition and application form (e.g., pastes, blocks, granules) is huge but most of them do not fulfill all main criteria for adequate bone substitutes: osteogenesis, osteoinductivity, osteoconductivity and osseointegration. Only autologous bone seems to comply with those demands (4).
In this perspective the research article from Laurenti et al. (5) exactly responds to this issue. Especially, if looking for alternatives to traditional inorganic scaffolds with limited injectability and biodegradability (2,6). Laurenti et al. presented a material formulation comprising three topics being currently of crucial interest within the research area of bone tissue engineering (TE): two-dimensional (2D)-nanomaterials, hydrogels and magnesium phosphate (MgP) minerals. In brief, MgP-nanosheets were fabricated and successfully formed a physically crosslinked long-term stable hydrogel which was injectable through a needle with a very small inner diameter. Without shearing the gel, it behaved like a solid material (Figure 1), whereby the application of a mechanical load resulted in shear-thinning with a dramatic increase of injectability. The authors proved that this material had osteogenic properties and could accelerate bone healing and osseointegration though it contained no osteoinductive supplements like cells or growth factors.
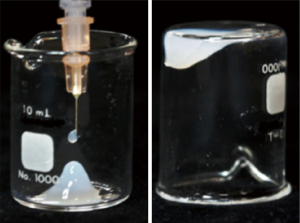
Nanomaterials are of great interest in many different research areas as they have unique size-dependent properties (7). In biomaterial engineering, they offer a good possibility to mimic the hierarchical architecture of native extracellular matrix (e.g., nanofibers) or might be used for the delivery and release of small bioactive molecules such as growth factors or DNA (2). Hydrogels are physically or chemically crosslinked polymer chains that are able to bind high amounts of water depending on properties like their mesh size (8). They can be designed in a way such that they are similar to native extracellular matrix where cells feel comfortable in (9). A lot of different hydrogel formulations have been shown to be biocompatible and they can be synthesized on the basis of biopolymers such as gelatin and alginate (8).
Recently, MgP minerals have attracted a broad interest in terms of bone regeneration as a competitive alternative for CaP ceramics. They are likewise biocompatible (10), but hydrated products of MgP-cements have a higher degradation potential (10,11), as released Mg2+-ions are known to oppress the precipitation of worse soluble re-precipitates (11,12). Magnesium further has an impact on bone remodeling: it was shown to promote osteoblast differentiation and inhibit osteoclastogenesis in a dose-dependent manner (13). Magnesium represents 1.6 wt.% of human bone (14) which contains 50–60% of the total magnesium amount in the body (15). The synthesis of the MgP-nanosheets represents a major advantage within the discussed publication because a simple precipitation route was chosen. Usually, the synthesis of 2D-nanomaterials is linked to time-consuming processes with toxic and expensive chemicals or high pressures and temperatures (e.g., exfoliation). Here, the raw materials simply consisted of brucite [Mg(OH)2] dissolved in phosphoric acid (H3PO4) and merged with sodium hydroxide (NaOH) solution. Mixing both, a MgP colloidal suspension with the precipitate MgxNay(HPO4)z(PO4)T·nH2O was obtained. Figure 2A depicts the phase diagram within the system Mg(OH)2-NaOH-H3PO4, but stable colloidal suspensions only formed in the red area whereat formulation A (molar ratio Mg(OH)2/NaOH/H3PO4 =0.13/0.52/0.3) and B (molar ratio =0.18/0.45/0.37) showed long-term stabilities of 2–4 years without mineral phase transformations. In those cases, the mineral composition of the precipitate was close to newberyite (MgHPO4·3H2O) in terms of the Mg/P ratio (1–1.15) and crystal water amount (3≤n≤4). The resulting crystals had a laminar morphology with high aspect ratio and a thickness of 4–7 nm (Figure 2B).
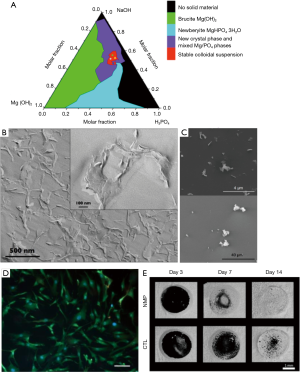
MgP-nanocrystals showed attractive interactions with both negatively and positively charged glass surfaces which confirmed the presence of both charges within the crystals (Figure 2C). This enabled the formation of physically crosslinked gels with water through electrostatic and van der Waals interactions. Within the gel, the nanoparticles formed a three-dimensional (3D)-honeycomb network with partially overlapping aggregates (Figure 2B). Gels with a MgP-nanosheet content of 5–10 wt.% behaved like thixotropic fluids with shear-thinning effect with high viscosities at low shear rates and vice versa which is beneficial for injectability and minimally invasive surgeries. Notably, the gel was injectable through a small gauge insulin needle by applying 9–18 N at a velocity of 0.3 mm/min.
In vitro studies suggested good cell viability of human fibroblasts (Figure 2D) especially for colloidal suspensions based on formulation B which had a pH close to physiological conditions (7.8). In differentiating osteoblasts from mouse bone marrow cells, this suspension induced the upregulation of osteogenesis related genes such as alkaline phosphatase (early osteogenesis) and osteocalcin (bone mineralization). Simultaneously, an increase in nanocrystal porosity during cell culture indicated their bioresorbability. Using a rat tibia model, an accelerated bone healing and osseointegration was demonstrated. Micro-computed tomography (µCT) scans revealed that the defect treated with MgP-nanosheet colloidal suspension was completely filled with new bone already 2 weeks after surgery compared to the control group. Simultaneously, a dense bone-implant contact was supported (Figure 2E). Histology and histomorphometric analysis confirmed these observations, as osteoblast differentiation, collagen synthesis and mineralization were promoted by the new bone void filler. At the same time, the in vivo resorption of the gel seemed to be fully accomplished after 3 days.
In summary, the material formulation presented by Laurenti et al. (5) seems to have several superior characteristics compared to conventional synthetic bone substitutes, e.g., an enhanced bone healing capacity by activating both osteoblasts and osteoclasts as well as thixotropic paste behavior for minimal invasive application through thin needles. This is in our view a significant step forward and a paradigm shift in developing novel bone replacement formulations, e.g., for orthopedic or craniofacial applications.
Acknowledgements
Funding: None.
Footnote
Provenance and Peer Review: This article was commissioned and reviewed by the Section Editor Zhantao Deng (Department of Orthopedics, Jinling Hospital, Medical School of Nanjing University, Nanjing, China).
Conflicts of Interest: Both authors have completed the ICMJE uniform disclosure form (available at http://dx.doi.org/10.21037/amj.2017.04.01). The authors have no conflicts of interest to declare.
Ethical Statement: The authors are accountable for all aspects of the work in ensuring that questions related to the accuracy or integrity of any part of the work are appropriately investigated and resolved.
Open Access Statement: This is an Open Access article distributed in accordance with the Creative Commons Attribution-NonCommercial-NoDerivs 4.0 International License (CC BY-NC-ND 4.0), which permits the non-commercial replication and distribution of the article with the strict proviso that no changes or edits are made and the original work is properly cited (including links to both the formal publication through the relevant DOI and the license). See: https://creativecommons.org/licenses/by-nc-nd/4.0/.
References
- Giannoudis PV, Dinopoulos H, Tsiridis E. Bone substitutes: an update. Injury 2005;36:S20-7. [Crossref] [PubMed]
- Holzapfel BM, Reichert JC, Schantz JT, et al. How smart do biomaterials need to be? A translational science and clinical point of view. Adv Drug Deliv Rev 2013;65:581-603. [Crossref] [PubMed]
- Dorozhkin SV. Bioceramics of calcium orthophosphates. Biomaterials 2010;31:1465-85. [Crossref] [PubMed]
- Moore WR, Graves SE, Bain GI. Synthetic bone graft substitutes. ANZ J Surg 2001;71:354-61. [Crossref] [PubMed]
- Laurenti M, Al Subaie A, Abdallah MN, et al. Two-Dimensional Magnesium Phosphate Nanosheets Form Highly Thixotropic Gels That Up-Regulate Bone Formation. Nano Lett 2016;16:4779-87. [Crossref] [PubMed]
- Bohner M, Gbureck U, Barralet JE. Technological issues for the development of more efficient calcium phosphate bone cements: a critical assessment. Biomaterials 2005;26:6423-9. [Crossref] [PubMed]
- Borm PJ, Robbins D, Haubold S, et al. The potential risks of nanomaterials: a review carried out for ECETOC. Part Fibre Toxicol 2006;3:11. [Crossref] [PubMed]
- Van Vlierberghe S, Dubruel P, Schacht E. Biopolymer-based hydrogels as scaffolds for tissue engineering applications: a review. Biomacromolecules 2011;12:1387-408. [Crossref] [PubMed]
- Jungst T, Smolan W, Schacht K, et al. Strategies and Molecular Design Criteria for 3D Printable Hydrogels. Chem Rev 2016;116:1496-539. [Crossref] [PubMed]
- Ostrowski N, Roy A, Kumta PN. Magnesium Phosphate Cement Systems for Hard Tissue Applications: A Review. ACS Biomater Sci Eng 2016;2:1067-1083. [Crossref]
- Kanter B, Geffers M, Ignatius A, et al. Control of in vivo mineral bone cement degradation. Acta Biomater 2014;10:3279-87. [Crossref] [PubMed]
- Bohner M. Reactivity of calcium phosphate cements. J Mater Chem 2007;17:3980-6. [Crossref]
- Wu L, Feyerabend F, Schilling AF, et al. Effects of extracellular magnesium extract on the proliferation and differentiation of human osteoblasts and osteoclasts in coculture. Acta Biomater 2015;27:294-304. [Crossref] [PubMed]
- Boanini E, Gazzano M, Bigi A. Ionic substitutions in calcium phosphates synthesized at low temperature. Acta Biomater 2010;6:1882-94. [Crossref] [PubMed]
- Cashman KD, Flynn A. Optimal nutrition: calcium, magnesium and phosphorus. Proc Nutr Soc 1999;58:477-87. [Crossref] [PubMed]
Cite this article as: Brückner T, Gbureck U. Nano-magnesium phosphate hydrogels: efficiency of an injectable and biodegradable gel formulation towards bone regeneration. AME Med J 2017;2:51.