Bombesin antagonist based radiotherapy of prostate cancer combined with WST-11 vascular targeted photodynamic therapy
Kim et al. (1) from Memorial Sloan-Kettering Cancer Center investigated the possibility to enhance the effect of radionuclide therapy by vascular-targeted photodynamic (VTP) therapy in an experimental prostate cancer animal model. They used bombesin-antagonist peptide as a targetor which is binding gastrin releasing peptide receptor (GRPr) and it was labeled with high energy beta-emitting radionuclide 90Y and the radionuclide was linked with DOTA-chelate. This peptide receptor radionuclide therapy (PRRT) compound, 90Y-DOTA-AR (bombesin antagonist) radiopeptide was followed by therapy with WST-11 (Weizmann STeba-11 drug; a negatively charged water-soluble palladium-bacteriochlorophyll derivative, Tookad® Soluble) application. This VTP approach generates reactive oxygen/nitrogen species within tumor blood vessels, resulting in their instantaneous destruction and causes rapid tumor necrosis. Radionuclide emitting charged particle forms Cherenkov luminescence imaging (CLI) which can be used to monitor the retention of the accumulated 90Y-PRRT. In this work, they demonstrated that the instantaneous arrest of tumor vasculature trapped radiopharmaceuticals in the vicinity of the tumor and thus improved the efficacy of targeted radiotherapy (1).
They used two GRPr positive prostate cancer xenograft models derived from PC-3 and VCaP cell lines. The animals were randomly assigned to four different groups, including sham control (saline and illumination only), VTP, 90Y-DOTA-AR, and combination of 90Y-DOTA-AR and VTP (the design is shown in Figure 1). The uptake of radioisotopes was monitored by CLI. CLI of 90Y-PRRT demonstrated statistically significant longer retention of radiotracer within the VTP treated PC-3 xenografts compared with the non-VTP treated ones, and similar results were observed with VCaP xenografts. Also, when 90Y-PRRT was combined with VTP, tumor growth delay was significantly longer than for the control or the other therapy groups (Figure 2). This means, that tumor vascular arrest by VTP improves 90Y-DOTA-AR retention in the tumor microenvironment thereby enhancing therapeutic efficacy.
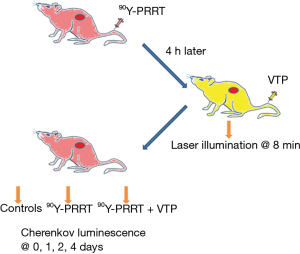
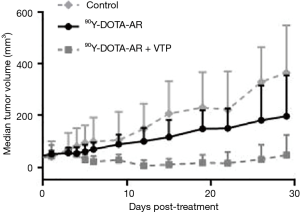
VTP is a new non-thermal ablation method involving systemic administration of the photosensitizing agent WST-11. Conventional photodynamic therapy agents are extravasated to the surrounding tissues mainly affecting the tumor cells (2), while WST-11 non-covalently binds to albumin and mainly targets the tumor vasculature (3). Permanent vascular arrest is limited to vessels smaller than 40 m, which is well suited to the tumor microenvironment (3).
GRPr, i.e., bombesin receptor subtype 2, is overexpressed in multiple human cancers at a high incidence rate (4), and all prostate cancers demonstrated receptor specific binding of radiolabeled bombesin in all tumors (n=30), whereas normal prostate and benign prostate hyperplasia displayed only minimal binding of bombesin (5). Radiolabeled bombesin analogs have been developed as targeting vectors for imaging and radionuclide therapy of tumors via specific binding to GRPr (6) and have been applied to clinical studies with radiolabeled bombesin based peptides in the imaging of metastasized prostate tumors with success (7). Importantly, one bombesin antagonist peptide, 111In-DOTA-AR (111In-DOTA-PEG4-D-Phe-Gln-Trp-Ala-Val-Gly-His-Sta-Leu-NH2), has demonstrated favorable affinity to GRPr and optimal uptake at 1–4 hours in PC-3 human prostate cancer xenografts (8).
This peptide DOTA-AR used here is a high-energy beta emitter 90Y (t1/2 =64 hours) which makes it suitable for non-invasive CLI, 90Y-DOTA-AR could act as a surrogate to investigate whether the arrest of tumor microvasculature in the target tissue enhances the radionuclide retention.
In summary, the initial experience with combinatorial sequential therapy using VTP with PRRT demonstrates very promising results. This has potential to improve cytotoxic effects in prostate cancers, because VTP does not show any serious photo-toxicity and its interval between drug injection and laser illumination is short. VTP provides an attractive option to decrease of fast washout of the accumulated radiopharmaceuticals from tumors.
Radionuclide therapies are effective in the treatment of prostate cancer, and they are part of current clinical practice especially in Europe and Australia. Two rather new methods, Lu-177-PSMA-617 and Ra-223 are shown to effectively improve survival (9,10). Combination of these with Cherenkov luminescence and vascular targeted therapy may improve therapy efficacy in metastatic tissues which are more radiation resistant.
Acknowledgements
Funding: None.
Footnote
Provenance and Peer Review: This article was commissioned and reviewed by the Section Editor Xiao Li (Department of Urology, The Affiliated Cancer Hospital of Jiangsu Province of Nanjing Medical University, Nanjing, China).
Conflicts of Interest: Both authors have completed the ICMJE uniform disclosure form (available at http://dx.doi.org/10.21037/amj.2017.09.01). The authors have no conflicts of interest to declare.
Ethical Statement: The authors are accountable for all aspects of the work in ensuring that questions related to the accuracy or integrity of any part of the work are appropriately investigated and resolved.
Open Access Statement: This is an Open Access article distributed in accordance with the Creative Commons Attribution-NonCommercial-NoDerivs 4.0 International License (CC BY-NC-ND 4.0), which permits the non-commercial replication and distribution of the article with the strict proviso that no changes or edits are made and the original work is properly cited (including links to both the formal publication through the relevant DOI and the license). See: https://creativecommons.org/licenses/by-nc-nd/4.0/.
References
- Kim K, Zhang H, La Rosa S, et al. Bombesin Antagonist-Based Radiotherapy of Prostate Cancer Combined with WST-11 Vascular Targeted Photodynamic Therapy. Clin Cancer Res 2017;23:3343-51. [Crossref] [PubMed]
- Juarranz A, Jaén P, Sanz-Rodríguez F, et al. Photodynamic therapy of cancer. Basic principles and applications. Clin Transl Oncol 2008;10:148-54. [Crossref] [PubMed]
- Mazor O, Brandis A, Plaks V, et al. WST11, a novel water-soluble bacteriochlorophyll derivative; cellular uptake, pharmacokinetics, biodistribution and vascular-targeted photodynamic activity using melanoma tumors as a model. Photochem Photobiol 2005;81:342-51. [Crossref] [PubMed]
- Reubi JC, Wenger S, Schmuckli-Maurer J, et al. Bombesin receptor subtypes in human cancers: detection with the universal radioligand (125)I-[D-TYR(6), beta-ALA(11), PHE(13), NLE(14)] bombesin(6-14). Clin Cancer Res 2002;8:1139-46. [PubMed]
- Markwalder R, Reubi JC. Gastrin-releasing peptide receptors in the human prostate: relation to neoplastic transformation. Cancer Res 1999;59:1152-9. [PubMed]
- Mansi R, Fleischmann A, Mäcke HR, et al. Targeting GRPR in urological cancers--from basic research to clinical application. Nat Rev Urol 2013;10:235-44. [Crossref] [PubMed]
- Kähkönen E, Jambor I, Kemppainen J, et al. In vivo imaging of prostate cancer using [68Ga]-labeled bombesin analog BAY86-7548. Clin Cancer Res 2013;19:5434-43. [Crossref] [PubMed]
- Abiraj K, Mansi R, Tamma ML, et al. Bombesin antagonist-based radioligands for translational nuclear imaging of gastrin-releasing peptide receptor-positive tumors. J Nucl Med 2011;52:1970-8. [Crossref] [PubMed]
- Rahbar K, Ahmadzadehfar H, Kratochwil C, et al. German Multicenter Study Investigating 177Lu-PSMA-617 Radioligand Therapy in Advanced Prostate Cancer Patients. J Nucl Med 2017;58:85-90. [Crossref] [PubMed]
- Oyen W, Sundram F, Haug AR, et al. Radium-223 Dichloride (Ra-223) for the Treatment of Metastatic Castration-resistant Prostate Cancer: Optimizing Clinical Practice in Nuclear Medicine Centers. J OncoPathol 2015;3:1-25. [Crossref]
Cite this article as: Kairemo K, Kangasmäki A. Bombesin antagonist based radiotherapy of prostate cancer combined with WST-11 vascular targeted photodynamic therapy. AME Med J 2017;2:149.