Challenging and opportunities in clinical implementation of circulating cardiac biomarkers in diabetes mellitus
Introduction
Type 2 diabetes mellitus (T2DM) is one of the most important non-communicable diseases the prevalence of which is progressively increasing worldwide and is associated with an occurrence of cardiovascular (CV) disease and CV mortality in a global scale (1,2). Globally, 415 million adults aged from 20 to79 years have T2DM and it is expecting up to 2025 the number of diabetics as will be 2-fold higher to today (3). The major risk factors for T2DM patients afflicted an increased risk of death are conventional predictors, such as abdominal obesity, hypertension, dyslipidemia, while other conditions including microvascular and systemic inflammation, endothelial dysfunction, altered cardiac and vascular reparation, cardiac and kidney fibrosis, are involved in the plaque formation, atherosclerosis acceleration, development of CV remodeling, kidney dysfunction and heart failure (4-6). Finally, T2DM contributing to CV mortality and morbidity yields a large economic burden for patients and their families, public health and national health care systems in either developed or developing countries (7-9). It has been suggested that the risk of progression from prediabetes to T2DM and the CV event occurrence can be evaluated by several biomarkers that affect various pathophysiological stages of natural evolution of the disease (10,11). Moreover, biomarker-guided therapy of T2DM can vigorously strength the management of the disease and improve the s strategy toward prevention of the CV complications (12,13). The aim of the narrative review is to summarize the knowledge with respect to clinical perspectives of the implementation of the circulating biomarkers to stratify T2DM patients at higher CV risk.
Methodology
The bibliographic database of life science and biomedical information MEDLINE, EMBASE, Medline (PubMed), the Web of Science, and the Cochrane Central were searched for English publications satisfying the key words of this study. We used the following key words [diabetes mellitus], [type 2 diabetes mellitus], [cardiovascular risk], [cardiovascular risk factors], [cardiac biomarkers]; [circulating biomarkers]; [prognosis]. Both authors independently evaluated the quality of the articles, correspondence to the main idea of the study, and constructed the final list of the references
The vicious cycle of T2DM and CV diseases
CV diseases and events are attributes of T2DM progression (14). It has been established that hyperglycemia and lipotoxicity contribute to numerous molecular and cellular mechanisms, such as impaired insulin signaling, accumulation of advanced glycated end-products, altered cell autophagy, activation of renin angiotensin aldosterone and sympatric systems, and signal transduction though several receptors (G-protein receptor kinase, and β-2 adrenergic receptors), which support the development of the oxidative stress, systemic and microvascular inflammation, endoplasmic reticulum and mitochondrial stress, neutrophil extracellular traps, and changes in myocardial and vascular structure (15-17). These common mechanisms are substantial for overlap in T2DM and conventional CV risk factors, such as hypertension, dyslipidemia, abdominal obesity, and smoking (18,19). Up-regulation in the inflammation, neurohumoral response and oxidative stress, and activation of the immune system in diabetics leads to microvascular inflammation, acceleration of atherosclerosis, cardiac and vascular remodeling, development of pro-thrombotic state and finally the occurrence of CV events including CV death, nonfatal myocardial infarction, non-fatal stroke, urgent hospitalization for unstable angina, life-threatening arrhythmias and heart failure (20-22). Figure 1 is reported the vicious cycle of T2DM and CV disease interplay. Thus, conventional CV risk factors, hyperglycaemia and metabolic abnormalities secondary to T2DM (lipotoxicity, insulin resistance, adipocytokine dysfunction), acceleration of atherosclerosis, cardiac hypertrophy and myocardial impairment, and vascular injury increases the risk of macrovascular and microvascular complications shaping the vicious cycle in T2DM (23,24).
CV biomarkers in T2DM
Whether T2DM patients has the same risk of premature death as those with established coronary heart disease and whether T2DM should be considered as equivalent to high and very high CV risk is not obvious and it is remaining under scientific debates now (25). Contemporary paradigm in T2DM management is based on the importance of point-to-care therapies and stratification of the patients at CV risk based on considerations including aging, comorbidities, stablished CV diseases, and biomarkers not merely for glycaemia control, but applying several target organ damage (26-28). Circulating biomarkers reflecting various pathophysiological stages of the evolution of T2FDM and its CV complications allow identifying a risk of the patents and predicting clinical outcomes for many cases (Figure 2). Current clinical recommendations from the American Heart Association, the American Diabetes Association and the European Society of Cardiology focus on the biomarkers of biomechanical stress, fibrosis and inflammation (26-28).
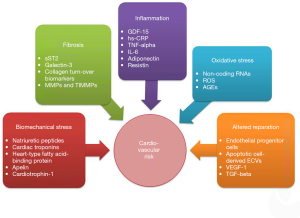
Biomechanical stress biomarkers
Natriuretic peptides (NPs)
NPs are well established biomarkers of biomechanical stress due to cardiac wall stretching, volume overload, myocardial ischemia/necrosis, inflammation and myocardial hypertrophy (29). Having a wide range of specific function in the regulation of water and electrolyte homeostasis, vasodilation, diuresis, proliferative and inflammatory responses, the family of NPs is involved in the adaptive molecular mechanisms counteracting to cardiac and vascular remodeling, kidney fibrosis, and endocrine dysfunction (30). Atrial, brain and endothelial types of NPs exert their biological effects through specific receptors and play a protective role against the development of CV remodeling, microvascular inflammation, renal and endothelial dysfunction, accelerating atherosclerosis, metabolic abnormalities (insulin resistance, lipid metabolism), adipose tissue and skeletal muscle dysfunction (31-33). In addition, atrial and brain NPs regulate tissue expressions of the pro-inflammatory genes and thereby mediate cardiac protective effect (34). Moreover, neprilysin gene expression in T2DM patients is under close control of NPs and this impact is driven through the epigenetically suppression of the H2AK5Ac, H2BK5Ac, H3K18Ac, and H4K8Ac histone acetylation (35). Yet, BNP is produced not merely cardiac myocytes, but adipocytes of white adipose tissue, and this fact explains why the circulating levels of NPs in obese patients and diabetics with abdominal obesity are higher to those who do not have obesity (36,37). Therefore, low expression of adipose tissue receptors for NPs was associated with abdominal obesity and can relate to epigenetic regulation (38). Overall, among T2DM patients NPs support insulin sensitivity and reduce white adipose tissue accumulation.
Previous clinical studies have shown the strong correlation between brain NP (BNP) and N-terminal fragment of BNP (NT-proBNP) and a risk of heart failure (HF) onset and admission (39-41). Elevated levels of NPs were found in T2DM patients with established CV disease regardless of HF presentation (42). In addition, increased levels of NPs predicted CV morbidity and mortality, myocardial infarction, stroke, HF occurrence and hospitalization due to HF and other CV events in T2DM individuals with recent coronary events (42,43). Interestingly, diabetics with coronary artery disease (CAD) who did not demonstrate declining NT-proBNP over 6 months had a significantly higher risk for CV mortality and HF development in comparison with those who demonstrated low levels of NT-proBNP for treatment period (43). Moreover, there were found strong interrelations between NT-proBNP plasma levels and a risk of premature death in T2DM patients having either HFrEF or HFpEF (44). In addition, NPs having a kidney clearance reflect a link between HF, mortality and renal disease. Indeed, the urine NT-pro-C-type of NP to creatinine ratio was more reproducible than the albumin to creatinine ratio and strongly associated with the presence of chronic kidney disease (45). These facts open out new perspectives to treat T2DM patients under serial monitoring of NPs, while recent The Diabetes Prevention Program has revealed significant racial and ethnic differences in the levels of NPs in follow-up (46). Thus, lower NPs levels in African-Americans to white, American-Indian, and Asian individuals require serious explanation to identify the cardio-metabolic implications of serial measure of NPs levels in the clinical trials in the future.
Cardiac troponins
High sensitive cardiac troponins are established biomarkers of biomechanical stress, myocardial ischemia and necrosis (47). Mild elevation of serum levels of cardiac troponins is common in T2DM patients due to leakage of cell troponin fraction through damaged membranes of cardiac myocytes or in result of an increase in membrane permeability (48). It has been found that severe hypoglycemia/hypoglycemia and multiple CV risk factors could associate with elevated hs-TnT levels (49,50). Even youth with T2DM has demonstrated higher levels of high-sensitive circulating troponin T (hs-cTnT) to healthy volunteers (51), but the clinical significance of this fact is unclear and requires to be investigated in further long-term studies. Although an increase in the cardiac troponin levels among diabetics without clinical signs and symptoms of HF was associated with the highest tumor necrosis factor (TNF) receptor tertile and adverse echocardiographic parameters, such as higher left ventricular mass, left atrial internal dimension and E/Em ratio, these relationships were non-existent after adjustment for body mass and age (51). However, a large proportion of T2DM patients has established CV disease or is at higher risk of CAD. Consequently, elevated plasma levels of high sensitive cardiac troponins can reflect either subclinical ischemia-related myocardial damage (≥99th percentile of reference level) or subclinical myocardial stress (<99th percentile of reference level) (52,53). It has been shown that increased levels of hs-cTnT in T2DM patients were correlated with persistent microalbuminuria, as well as elevated extracellular volume fraction, cardiac fibrosis, and diastolic dysfunction measured by CV magnetic resonance (54). Although hs-cTnT plasma level was found to be powerful independent predictor for newly diagnosed HF in T2DM patients with CAD (55), the discriminative potency of BNP/NT-proBNP was higher to hs-cTnT and combination of both biomarkers did not improve a prediction for HF (56-58). In contrast, among HFrEF patients with established T2DM combination of hs-cTnT and NT-proBNP demonstrated additive potency in CV risk prognosis (59). Finally, exercise-induced hs-cTnI elevations above the 99th percentile independently predicted higher mortality and CV events in T2DM patients regardless of HF presentation (60).
Biomarkers of inflammation and fibrosis
Soluble suppressor tumorigenicity-2
Soluble suppression of tumorigenicity 2 (sST2) is an interleukin-1 receptor family member that is produced by cardiac myocytes, endothelial cells and certain immune cells. sST2 is up-regulated in response to biomechanical myocardial stress, inflammation and ischemia and exerts cardio-protective and atheroprotective actions through inhibiting IL-33/ST2 signaling (61). In the myocardium sST2 reduces fibrosis, inflammation, hypertrophy and apoptosis of cardiac myocytes (62). Although sST2 lacks disease specificity, it has been incorporated into some clinical recommendations to use as predictive biomarker of CV death and the development of HF (63). In patients with acute and chronic HF elevated serum levels of sST2 strongly related to HF severity and poor clinical outcome (64). Peak concentrations and serial measurement of sST2 added the prognostic information to conventional HF biomarkers including NT-proBNP and hs-cTnT (65,66). Therefore, sST2 is independent predictor of the no-reflow phenomenon in STEMI patients underwent primary percutaneous coronary intervention (67) and all-cause mortality in individuals with atrial fibrillation (68).
Among general population elevated levels of sST2 was associated with not merely HF, but with T2DM, metabolic abnormalities, T2DM-related renal disease and atherosclerosis (69-71). In the patients having pre-diabetes and T2DM elevated concentrations of sST2 (>35 ng/mL) strongly predicted newly diagnosed HF and HF-related outcomes including hospital admission and death (72-74). To sum up, sST2 is now one of independent predictors of CV mortality, all-cause mortality, HF occurrence and HF-related outcomes in either general population or among T2DM patients.
Growth differential factor-15
Growth differentiation factor-15 (GDF-15) is a multifunctional cytokine that belongs to transforming growth factor beta superfamily (75). It is widely expressed on the various cells and contributes to anti-inflammatory and tissue protective properties (76). GDF-15 suppresses c-Jun N-terminal kinase, Bcl-2-associated death promoter and epidermal growth factor receptor signaling pathway and stimulates Smad/eNOS, and PI3K/AKT signaling mechanisms to maintain a survival of cardiac myocytes, adipocytes, and progenitor and mature endothelial cells (75).
Numerous CV diseases including CAD, atherosclerosis, peripheral artery disease, acute myocardial infarction, HF, and stroke were accompanied by elevated concentrations of the GDF-15 (77-79). It has been reported that the circulating levels of GDF-15 >3,812 pg/mL are clearly indicative for T2DM among patients without established CV disease (80,81). The results of the Malmö Diet and Cancer-Cardiovascular Cohort have shown that serum levels of the GDF-15 predicted newly diagnosed T2DM and positively respond to metformin (82-84). Interestingly, the GDF-15 was associated with impaired fasting glucose levels and insulin resistance in non-T2DM population (85). Therefore, elevated levels of the GDF-15 were found to be predictive of all-cause mortality, diabetes mellitus related renal disease, HF, and HF-related outcomes in T2DM (86-88). Thus, the measure of the serum levels of GDF-15 appears to be promising in both CV and metabolic risk stratification, while it is not clear whether GDF-15 will be more meaningful biomarker to sST2 or NPs in patients at risk of T2DM or among diabetics with established CV disease including HF. In addition, there is no evidence what multiple combinations based in GDF-15, sST2, NPs, and cardiac troponins will be more optimal for risk identification.
Circulating convention cardiac biomarkers and antidiabetic drugs
Dipeptidyl peptidase-4 inhibitors
The dipeptidyl peptidase-4 (DPP-4) inhibitors were found to have a significant variability in their CV safety. For instance, the results of post-hoc analyses of 2nd and 3rd phases of randomized placebo controlled trials depicted DPP-4 inhibitors (sitagliptin, saxagliptin, vildagliptin, linagliptin, and alogliptin) have shown a cardioprotective effect with a sustainable trend toward declining MACEs (89). In contrast, there were no significant differences between DPP-4 inhibitors and placebo in CV safety in the TECOS (Sitagliptin Cardiovascular Outcome Study) trial on sitagliptin, the EXAMINE (Cardiovascular Outcomes Study of Alogliptin in Subjects With Type 2 Diabetes and Acute Coronary Syndrome) trial on alogliptin, and the CAROLINA (Cardiovascular Outcome Study of Linagliptin Versus Glimepiride in Patients With Type 2 Diabetes) trial on linagliptin. The SAVOR-TIMI 53 (Saxagliptin Assessment of Vascular Outcomes Recorded in Patients With Diabetes Mellitus-Thrombolysis in Myocardial Infarction 53 trial) study has been reported that the treatment with saxagliptin versus placebo was associated with an increased risk or HF hospitalization (90-94). In addition, this increase in the risk was highest among patients with elevated levels of NPs, previous HF, or chronic kidney disease (90). Moreover, hs-TnT, NT-proBNP, and hs-CRP were related to a reduction in eGFR (<40%) and HF hospitalization in patients with T2DM (91). Thus, cardiac biomarker predicted the occurrence and the progression of newly diagnosed HF in patient population with established T2DM.
Sodium glucose co-transporter 2 (SGLT2) inhibitors
Previous clinical studies have revealed that sodium glucose co-transporter 2 inhibitors may reduce CV and HF risk in HFrEF patients either with T2DM or without it (95-97). Interestingly, circulating levels of cardiac biomarkers, such as (NT-proBNP, hs-TnI, sST2, and galectin-3, modestly increased over two-year period among patients with poor clinical outcomes, whereas SGLT2 inhibitor canagliflozin delayed the rise in serum levels of NT-proBNP and hs-TnI that was associated with improving prognosis (98). In the DEFINE-HF (Dapagliflozin Effects on Biomarkers, Symptoms and Functional Status in Patients with HF with Reduced Ejection Fraction) Trial the use of SGLT2 inhibitor dapagliflozin over 12 weeks did not lead to the decrease in NT-proBNP serum levels, but was associated with meaningful improvements in HF-related quality of life status (99). The CV effects of new SGLT2 inhibitor ertugliflozin has investigated in the VERTIS-CV trial (eValuation of ERTugliflozin effIcacy and Safety CardioVascular outcomes) trial, in which 30% drop in HF admission risk in the ertugliflozin arm versus placebo arm, was established (100). Whether serial measure of the levels of circulating cardiac biomarkers is effective in prediction of CV outcomes is not still reported. Overall, the treatment initiation with SGLT-2 inhibitors was associated with lower risk of death and HF admission in comparison with other antidiabetic drugs and their combinations among T2DM patients with pre-existing CAD or at high risk of CAD (101,102). In this context, novel biomarkers can be used to thoroughly evaluate the CV risk and predict HF-related outcomes in diabetics without established CV disease and HF (103).
Glucagon-like peptide-1 receptor agonists
In the meta-analysis of 34 clinical trials (n=14,464) there were no differences in CV safety within short-acting glucagon-like preptide-1 (GLP-1) exenatide and lixisenatide or long-acting (albiglutide, dulaglutide, once-weekly exenatide, liraglutide and taspoglutide) groups (104). GLP-1 receptor agonists did not reduce the risk for HF hospitalization in T2DM and their safety in patients with known HF remains equivocal (105). Whether cardiac biomarkers are effective in HF risk stratification is not completely understood (10).
Other biomarkers
Matrix metalloproteinases (MMPs)
The family of MMPs is a group of Zn-contained proteolytic enzymes the main biological function of which is extracellular matrix turnover (106). In T2DM MMPs implicate with the development and advance of diabetic-related complications including cardiomyopathy, atherosclerosis, nephropathy, retinopathy, authonomic and peripheral neuropathies, and microvascular angiopathy (107,108). MMP-2, MMP-3, and MMP-9 were found in elevated concentration in the peripheral blood among patients with T2DM having a higher risk of the microangiopathy and retinopathy (109,110). It has been suggested that these MMPs regulating function of immune cells (Treg, macrophages), angiogenesis, vascular remodeling, repair vascular activity and apoptosis through activity through PPAR-alpha/gamma and tissue inhibitor MMP-1-(TIMP-1)-mediated mechanisms and also via NF-κB and MAP kinase signaling pathways are able to modulate tissue susceptibility to ischemia and injury (111-113). In fact, MMPs could have a dual role in T2DM contributing to the occurrence of the cardiomyopathy, retinopathy, and vascular complications at the early stages of the disease, and modulating tissue protection at the late stages through suppression of vascular permeability capillary cell apoptosis, neovascularization and potentiation of mitochondrial stress and extracellular matrix remodeling. Overall, there is no convincing evidence that MMPs reduce the risk of CAD or HF and their expression/concentration could be concise biomarker of the disease progression.
Vascular endothelial growth factor
Vascular endothelial growth factor-1 (VEGF-1) is an important endogenous tissue protective factor contributing to angiogenesis, vascular function, proliferation and apoptosis (114). It has been found increased tissue expression and elevated circulating concentration of VEGF-1 among animals and patients having T2DM (115,116). VEGF-1 reduced production of pro-inflammatory cytokines (TNF-alpha, IL-6, IL-1-beta), monocyte chemotactic proteins-1, intercellular adhesion molecule-1 and mobilize proliferative activity of progenitor endothelial cells via phosphorylation of p65 Nuclear Factor Kappa B (NF-κB) and Extracellular signal Regulated Kinase (ERK) 1/2 (117). There is evidence of the fact that VEGF-1 directly activated several major trophic factors, such as TGF-beta, myocyte enhancer factor 2c, stromal-derived factor-1 and thereby influenced the progenitor cell cascade (118,119).
Numerous complications of T2DM (renal disease, retinopathy, microangiopathy, macroangiopathy, accelerating atherosclerosis) were associated with altered expression of the VEGF-1 and declined circulating levels of this peptide (120-122). Probably, elevated levels of VEGF-1 protect against the ischemia injury and the acceleration of atherosclerosis (123,124). Whether continuous monitoring of the circulating levels of VEGF-1 is useful to stratify patients having T2DM at higher risk of T2DM-related complications and adjust the therapy is not clear.
Collagen turnover biomarkers
Development and progression of T2DM is associated with remarkable loss of bone mass, increased CV risk and a risk of fragile (125). Therefore, the progression of atherosclerosis and ectopic calcifications including vascular and cardiac valve calcification accompany by altered profile of collagen turnover biomarkers (126). There are numerous data, which confirmed the predictive role of bone-related proteins (osteopontin, osteoprotegerin) and collagen turnover biomarkers (carboxyl-terminal peptide of procollagen type I, carboxyl-terminal telopeptide of collagen type I, and amino-terminal peptide of procollagen type III) in CV mortality, HF occurrence and progression, chronic kidney disease, and MACEs (125,127-130).
Serum levels of the bone resorption marker (C-terminal cross-linked telopeptide) and the bone formation markers (osteocalcin and procollagen type 1 amino terminal propeptide) were significantly lower, whereas concentrations of both osteoprotegerin and sclerostin were higher in T2DM patients in comparison with healthy volunteers (131). These finding indicate that the collagen homeostasis is critically important for the development and the progression of T2DM and that control for glucose metabolism may relate to osteopenia and CV risk through altered production of bone-related proteins, such as osteopontin, osteocalcin, and osteoprotegerin (132). Another meta-analysis consisting of 611 clinical studies has shown that C-terminal cross-link of collagen, insulin-like growth factor-1, and sclerostin may potentially predict the fractures among T2DM patients (133). In fact, some investigators reported that T2DM-related microvascular disease and CV risk were associated with the impaired profile of collagen turn-over biomarkers, circulating levels of osteopontin and osteoprotegerin, but after adjustment for glomerular filtration rate these interrelations were sufficiently diminished or even completely missed (134-136). However, large longitudinal studies are needed to elucidate whether collagen turn-over biomarkers are independent predictors of CV events and T2DM-related complications.
The number and function of the endothelial progenitor cells (EPCs)
EPCs are a heterogeneous population of endothelial precursors originated from bone marrow stem cells or peripheral tissue resident cells and having pro-angiogenic and tissue protective abilities (137). The natural evolution of T2DM is associated with decrease in the number of the EPCs and weak their functional ability to differentiate into mature endothelial cells and mediate the vascular reparation, which was called EPC dysfunction (138-140). Lowered number of circulating EPCs strongly correlated with CV risk and predicted MACEs, CV diseases including myocardial infarction and HF, and T2DM-related complications (141-143). Restoring the number and function of EPCs was associated with improvement in NO bioavailability, endothelial function, neovascularization, and decrease in the risk of poor clinical outcomes in T2DM (144,145).
Thus, the number of EPCs is promising biomarker with predictive ability and potential therapeutic target, whereas functional abnormalities of EPCs have demonstrated more close relation to poor clinical outcomes, when compared to merely measure of the number of EPCs, but difficulties to implementation of this approach in the routine laboratory practice make investigators to use a measure of EPC count in peripheral blood (146,147). Future investigations could affect EPC alterations as predictive biomarkers of long-term outcomes and mortality among T2DM.
Endothelial cell derived extracellular vesicles (EVs)
Endothelial cell derived EVs are produced by progenitor and mature endothelial cells and are a crucial element in cell-to-cell cooperation and transportation of several molecules (proteins, lipids, growth factors and hormones, active molecules, non-coding RNAs) to target cells (148,149). There is a large body of evidence of the fact that T2DM development corresponded to altered profile of circulating EVs (150,151). For instance, metabolic syndrome in patients without known CV disease is associated with increase in the levels of endothelial cell derived EVs, but patients T2DM, morbid obesity, CAD, and HF demonstrated lower levels of EVs originated from endothelial cells to those who did not established CV or metabolic disease (152-154). Moreover, some previous meta-analysis have revealed that increased levels of apoptotic endothelial cell derived EVs and decreased mature endothelial cell derived EVs were associated with higher CV risk and the risk of occurrence and progression of CAD and HF (155-157). EVs are promising biomarkers which can be either predictor or targets for innovative therapeutic approaches in the future.
Biomarkers related to CV rehabilitation, exercise or lifestyle modification
Cardiac rehabilitation reduces all-cause and CV mortality in T2DM patients with established CV disease (158). Modest improvements in long-term survival among diabetics with known CV disease suggest that cardiorespiratory fitness programs may require optimization with longitudinal cardiac biomarker measure. For instance, the coincidence of completion of exercise training with the decrease in the levels of NPs or sST2 would be a good confirmation of success in cardiac rehabilitation. Although the acute effects of continuous moderate intensity aerobic exercise and high intensity interval aerobic exercise on endothelial function, arterial stiffness, cardiac remodeling in obese patients having prediabetes and T2DM were widely investigated, the remote effects of cardiorespiratory fitness programs among these individuals under control of cardiac biomarker measure need to be evaluated in the future. However, there is evidence regarding that the concomitant reduction in adiposity or circulating biomarkers in patients having prediabetes or T2DM after exercise training program for 3-month period was associated with serious benefits in cardiometabolic health including beneficial effects on plasma lipids and fasting glucose (159-161). The STABILITY (Stabilization of Atherosclerotic Plaque by Initiation of Darapladib Therapy) study has been revealed strong association between decrease in all-cause/CV mortality and greater physical activity in the subgroup of patients at higher risk estimated by the ABC-CHD (Age, Biomarkers, Clinical-Coronary Heart Disease) risk score (162). Yet, life style modification and Mediterranean diet was associated with lower mortality in patients with CV disease, but not those who had T2DM without established CAD (162). In this context, circulating cardiac biomarkers could individualize non-pharmacological approach in those with the highest mortality risk. Probably, other biomarkers reflecting the endothelial function and inflammation will be useful for prediction of the risk of future CV events in diabetics (163,164). Large multicentre clinical trials are necessary to confirm these assumptions.
Future perspectives
The discovery of new biomarkers appears to be promising, while a lack of longitudinal investigations with aim to compare face-to-face conventional and new biomarkers make use speculative approaches to explanation of advantages and challenging. Perhaps, multiple markers models will be more acceptable to stratify at CV risk T2DM patients. In addition, there is a need to evaluate whether conventional and novel biomarkers could be powerful prognostic predictor for clinical outcomes in T2DM patients after MACEs. Large clinical trials are required to be planned and executed in the future to clearly elucidate what biomarker models are most optimal for this matter.
The limitations and quality of the articles reviewed
The limitations of the narrative review were a search of the article written English and a lack of blinded evaluation of the quality of them.
Conclusions
There is a wide range of circulating cardiac biomarkers that were widely investigated and incorporated into diagnostic and predictive models for patients at higher risk of CV disease and events. Conventional approach is based on the use of NPs and cardiac troponins in routine clinical practice, whereas there is no evidence that these biomarkers have equal diagnostic and predictive values for patients with prediabetes and established T2DM.
Acknowledgments
Funding: None.
Footnote
Peer Review File: Available at https://amj.amegroups.com/article/view/10.21037/amj-20-147/prf
Conflicts of Interest: Both authors have completed the ICMJE uniform disclosure form (available at https://amj.amegroups.com/article/view/10.21037/amj-20-147/coif). The authors have no conflicts of interest to declare.
Ethical Statement: The authors are accountable for all aspects of the work in ensuring that questions related to the accuracy or integrity of any part of the work are appropriately investigated and resolved.
Open Access Statement: This is an Open Access article distributed in accordance with the Creative Commons Attribution-NonCommercial-NoDerivs 4.0 International License (CC BY-NC-ND 4.0), which permits the non-commercial replication and distribution of the article with the strict proviso that no changes or edits are made and the original work is properly cited (including links to both the formal publication through the relevant DOI and the license). See: https://creativecommons.org/licenses/by-nc-nd/4.0/.
References
- Benjamin EJ, Virani SS, Callaway CW, et al. Heart disease and stroke Statistics-2018 update: a report from the American heart association. Circulation 2018;137:e67-e492. [Crossref] [PubMed]
- Tao Z, Shi A, Zhao J. Epidemiological Perspectives of Diabetes. Cell Biochem Biophys 2015;73:181-5. [Crossref] [PubMed]
- Zheng Y, Ley S, Hu F. Global aetiology and epidemiology of type 2 diabetes mellitus and its complications. Nat Rev Endocrinol 2018;14:88-98. [Crossref] [PubMed]
- Balakumar P, Maung-U K, Jagadeesh G. Prevalence and prevention of cardiovascular disease and diabetes mellitus. Pharmacol Res 2016;113:600-9. [Crossref] [PubMed]
- Zimmet P, Alberti KG, Magliano DJ, et al. Diabetes mellitus statistics on prevalence and mortality: facts and fallacies. Nat Rev Endocrinol 2016;12:616-22. [Crossref] [PubMed]
- Koye DN, Magliano DJ, Nelson RG, et al. The Global Epidemiology of Diabetes and Kidney Disease. Adv Chronic Kidney Dis 2018;25:121-32. [Crossref] [PubMed]
- Xu Z, Yu D, Yin X, et al. Socioeconomic status is associated with global diabetes prevalence. Oncotarget 2017;8:44434-9. [Crossref] [PubMed]
- Glovaci D, Fan W, Wong ND. Epidemiology of Diabetes Mellitus and Cardiovascular Disease. Curr Cardiol Rep 2019;21:21. [Crossref] [PubMed]
- Xu G, Liu B, Sun Y, et al. Prevalence of diagnosed type 1 and type 2 diabetes among US adults in 2016 and 2017: population based study. BMJ 2018;362:k1497. [Crossref] [PubMed]
- Berezin AE. Cardiac biomarkers in diabetes mellitus: New dawn for risk stratification. Diabetes Metab Syndr 2017;11:S201-S208. [Crossref] [PubMed]
- Johar DR, Bernstein LH. Biomarkers of stress-mediated metabolic deregulation in diabetes mellitus. Diabetes Res Clin Pract 2017;126:222-9. [Crossref] [PubMed]
- Lees T, Nassif N, Simpson A, et al. Recent advances in molecular biomarkers for diabetes mellitus: a systematic review. Biomarkers 2017;22:604-13. [Crossref] [PubMed]
- Berezin AE, Berezin AA. Circulating Cardiac Biomarkers in Diabetes Mellitus: A New Dawn for Risk Stratification-A Narrative Review. Diabetes Ther 2020;11:1271-91. [Crossref] [PubMed]
- Alvarez CA, Lingvay I, Vuylsteke V, et al. Cardiovascular Risk in Diabetes Mellitus: Complication of the Disease or of Antihyperglycemic Medications. Clin Pharmacol Ther 2015;98:145-61. [Crossref] [PubMed]
- Kenny HC, Abel ED. Heart Failure in Type 2 Diabetes Mellitus. Circ Res 2019;124:121-41. [Crossref] [PubMed]
- Petrie JR, Guzik TJ, Touyz RM. Diabetes, Hypertension, and Cardiovascular Disease: Clinical Insights and Vascular Mechanisms. Can J Cardiol 2018;34:575-84. [Crossref] [PubMed]
- Berezin A. Neutrophil extracellular traps: The core player in vascular complications of diabetes mellitus. Diabetes Metab Syndr 2019;13:3017-23. [Crossref] [PubMed]
- Berezin AE, Berezin AA, Lichtenauer M. Emerging roleof adipocyte dysfunction in inducing heart failure among obese patients with prediabetes and known diabetes mellitus. Front Cardiovasc Med 2020;7:583175. [Crossref] [PubMed]
- Pop-Busui R, Braffett BH, Zinman B, et al. Cardiovascular Autonomic Neuropathy and Cardiovascular Outcomes in the Diabetes Control and Complications Trial/Epidemiology of Diabetes Interventions and Complications (DCCT/EDIC) Study. Diabetes Care 2017;40:94-100. [Crossref] [PubMed]
- Sposito AC, Berwanger O, de Carvalho LSF, et al. GLP-1RAs in type 2 diabetes: mechanisms that underlie cardiovascular effects and overview of cardiovascular outcome data. Cardiovasc Diabetol 2018;17:157. [Crossref] [PubMed]
- Holvoet P. Relations between metabolic syndrome, oxidative stress and inflammation and cardiovascular disease. Verh K Acad Geneeskd Belg 2008;70:193-219. [PubMed]
- Tadic M, Cuspidi C. Type 2 diabetes mellitus and atrial fibrillation: From mechanisms to clinical practice. Arch Cardiovasc Dis 2015;108:269-76. [Crossref] [PubMed]
- Jahangiri-Noudeh Y, Akbarpour S, Lotfaliany M, et al. Trends in cardiovascular disease risk factors in people with and without diabetes mellitus: a Middle Eastern cohort study. PLoS One 2014;9:e112639. [Crossref] [PubMed]
- Zitouni K, Steyn M, Earle KA. Residual renal and cardiovascular disease risk in conventionally-treated patients with type 2 diabetes: the potential of non-traditional biomarkers. Minerva Med 2018;109:103-15. [PubMed]
- Katsiki N, Banach M, Mikhailidis DP. Is type 2 diabetes mellitus a coronary heart disease equivalent or not? Do not just enjoy the debate and forget the patient!. Arch Med Sci 2019;15:1357-64. [Crossref] [PubMed]
- Davies MJ, D'Alessio DA, Fradkin J, et al. Management of Hyperglycemia in Type 2 Diabetes, 2018. A Consensus Report by the American Diabetes Association (ADA) and the European Association for the Study of Diabetes (EASD). Diabetes Care 2018;41:2669-701. [Crossref] [PubMed]
- Seferovic PM, Ponikowski P, Anker SD, et al. Clinical practice update on heart failure 2019: pharmacotherapy, procedures, devices and patient management. An expert consensus meeting report of the Heart Failure Association of the European Society of Cardiology. Eur J Heart Fail 2019;21:1169-86. [Crossref] [PubMed]
- Pieske B, Tschöpe C, de Boer RA, et al. How to diagnose heart failure with preserved ejection fraction: the HFA-PEFF diagnostic algorithm: a consensus recommendation from the Heart Failure Association (HFA) of the European Society of Cardiology (ESC). Eur Heart J 2019;40:3297-317. [Crossref] [PubMed]
- Feng Y, Wang D, Bi H, et al. The role of natriuretic peptides in diabetes and its complications. Biomed Pharmacother 2016;84:1826-32. [Crossref] [PubMed]
- Berezin AE. Biomarkers for cardiovascular risk in patients with diabetes. Heart 2016;102:1939-41. [Crossref] [PubMed]
- Verboven K, Hansen D, Jocken JWE, et al. Natriuretic peptides in the control of lipid metabolism and insulin sensitivity. Obes Rev 2017;18:1243-59. [Crossref] [PubMed]
- Zois NE, Bartels ED, Hunter I, et al. Natriuretic peptides in cardiometabolic regulation and disease. Nat Rev Cardiol 2014;11:403-12. [Crossref] [PubMed]
- Moro C. Targeting cardiac natriuretic peptides in the therapy of diabetes and obesity. Expert Opin Ther Targets 2016;20:1445-52. [Crossref] [PubMed]
- Kerkelä R, Ulvila J, Magga J. Natriuretic Peptides in the Regulation of Cardiovascular Physiology and Metabolic Events. J Am Heart Assoc 2015;4:e002423. [Crossref] [PubMed]
- Malek V, Sharma N, Gaikwad AB. Histone acetylation regulates natriuretic peptides and neprilysin gene expressions in diabetic cardiomyopathy and nephropathy. Curr Mol Pharmacol 2019;12:61-71. [Crossref] [PubMed]
- Kaisanlahti A, Glumoff T. Browning of white fat: agents and implications for beige adipose tissue to type 2 diabetes. J Physiol Biochem 2019;75:1-10. [Crossref] [PubMed]
- Coué M, Barquissau V, Morigny P, et al. Natriuretic peptides promote glucose uptake in a cGMP-dependent manner in human adipocytes. Sci Rep 2018;8:1097. [Crossref] [PubMed]
- Gruden G, Landi A, Bruno G. Natriuretic peptides, heart, and adipose tissue: new findings and future developments for diabetes research. Diabetes Care 2014;37:2899-908. [Crossref] [PubMed]
- Pascual-Figal DA, Domingo M, Casas T, et al. Usefulness of clinical and NT-proBNP monitoring for prognostic guidance in destabilized heart failure outpatients. Eur Heart J 2008;29:1011-8. [Crossref] [PubMed]
- Verdiani V, Ognibene A, Rutili MS, et al. NT-ProBNP reduction percentage during hospital stay predicts long-term mortality and readmission in heart failure patients. J Cardiovasc Med (Hagerstown) 2008;9:694-9. [Crossref] [PubMed]
- Bettencourt P, Azevedo A, Pimenta J, et al. N-terminal-pro-brain natriuretic peptide predicts outcome after hospital discharge in heart failure patients. Circulation 2004;110:2168-74. [Crossref] [PubMed]
- Wolsk E, Claggett B, Diaz R, et al. Increases in natriuretic peptides precede heart failure hospitalization in patients with a recent coronary event and type 2 diabetes mellitus. Circulation 2017;136:1560-2. [Crossref] [PubMed]
- Jarolim P, White WB, Cannon CP, et al. Serial measurement of natriuretic peptides and cardiovascular outcomes in patients with type 2 diabetes in the EXAMINE Trial. Diabetes Care 2018;41:1510-5. [Crossref] [PubMed]
- Kristensen SL, Mogensen UM, Jhund PS, et al. Clinical and Echocardiographic Characteristics and Cardiovascular Outcomes According to Diabetes Status in Patients With Heart Failure and Preserved Ejection Fraction: A Report From the I-Preserve Trial (Irbesartan in Heart Failure With Preserved Ejection Fraction). Circulation 2017;135:724-35. [Crossref] [PubMed]
- Prickett TCR, Lunt H, Warwick J. at all. Urinary Amino-Terminal Pro-C-Type Natriuretic Peptide: A Novel Marker of Chronic Kidney Disease in Diabetes. Clin Chem 2019;65:1248-57. [Crossref] [PubMed]
- Gupta DK, Walford GA, Ma Y, et al. Racial/ethnic differences in circulating natriuretic peptide levels: The Diabetes Prevention Program. PLoS One 2020;15:e0229280. [Crossref] [PubMed]
- Zhong B, Wang Y, Zhang G. History of diabetes mellitus is associated with elevated cardiac troponin I levels in patients with chest pain but no coronary heart disease. Cardiol J 2016;23:149-54. [Crossref] [PubMed]
- Bashir A, Azharuddin M, Rashid I, et al. Predictors of cardiomyopathy in patients with type-2 diabetes mellitus with and without cardiovascular complications: A cross-sectional study. Diabetes Res Clin Pract 2019;154:90-100. [Crossref] [PubMed]
- Rezende PC, Everett BM, Brooks MM, et al. Hypoglycemia and elevated troponin in patients with diabetes and coronary artery disease. J Am Coll Cardiol 2018;72:1778-86. [Crossref] [PubMed]
- Odum EP, Young EE. Elevated cardiac troponin I, creatine kinase and myoglobin and their relationship with cardiovascular risk factors in patients with type 2 diabetes. Diabetes Metab Syndr 2018;12:141-5. [Crossref] [PubMed]
- Gidding SS, Bacha F, Bjornstad P, et al. Cardiac Biomarkers in Youth with Type 2 Diabetes Mellitus: Results from the TODAY Study. J Pediatr 2018;192:86-92.e5. [Crossref] [PubMed]
- Everett BM, Brooks MM, Vlachos HE, et al. Troponin and cardiac events in stable ischemic heart disease and diabetes. N Engl J Med 2015;373:610-20. [Crossref] [PubMed]
- Li J, Zhao Y, Zhou N, et al. Dexmedetomidine Attenuates Myocardial Ischemia-Reperfusion Injury in Diabetes Mellitus by Inhibiting Endoplasmic Reticulum Stress. J Diabetes Res 2019;2019:7869318. [Crossref] [PubMed]
- Swoboda PP, McDiarmid AK, Erhayiem B, et al. Diabetes mellitus, microalbuminuria, and subclinical cardiac disease: identification and monitoring of individuals at risk of heart failure. J Am Heart Assoc 2017;6:e005539. [Crossref] [PubMed]
- Lepojärvi ES, Piira OP, Kiviniemi AM, et al. Usefulness of Highly Sensitive Troponin as a Predictor of Short-Term Outcome in Patients With Diabetes Mellitus and Stable Coronary Artery Disease (from the ARTEMIS Study). Am J Cardiol 2016;117:515-21. [Crossref] [PubMed]
- Ohkuma T, Jun M, Woodward M, et al. Cardiac Stress and Inflammatory Markers as Predictors of Heart Failure in Patients With Type 2 Diabetes: The ADVANCE Trial. Diabetes Care 2017;40:1203-9. [Crossref] [PubMed]
- Wong YK, Cheung CYY, Tang CS, et al. High-sensitivity troponin I and B-type natriuretic peptide biomarkers for prediction of cardiovascular events in patients with coronary artery disease with and without diabetes mellitus. Cardiovasc Diabetol 2019;18:171. [Crossref] [PubMed]
- Lindholm D, Lindbäck J, Armstrong PW, et al. Biomarker-based risk model to predict cardiovascular mortality in patients with stable coronary disease. J Am Coll Cardiol 2017;70:813-26. [Crossref] [PubMed]
- Rørth R, Jhund PS, Kristensen SL, et al. The prognostic value of troponin T and N-terminal pro B-type natriuretic peptide, alone and in combination, in heart failure patients with and without diabetes. Eur J Heart Fail 2019;21:40-9. [Crossref] [PubMed]
- Aengevaeren VL, Hopman MTE, Thompson PD, et al. Exercise-Induced Cardiac Troponin I Increase and Incident Mortality and Cardiovascular Events. Circulation 2019;140:804-14. [Crossref] [PubMed]
- Pascual-Figal DA, Lax A, Perez-Martinez MT, et al. Clinical relevance of sST2 in cardiac diseases. Clin Chem Lab Med 2016;54:29-35. [Crossref] [PubMed]
- Dieplinger B, Mueller T. Soluble ST2 in heart failure. Clin Chim Acta 2015;443:57-70. [Crossref] [PubMed]
- AbouEzzeddine OF, McKie PM, Scott CG, et al. Biomarker-based risk prediction in the community. Eur J Heart Fail 2016;18:1342-50. [Crossref] [PubMed]
- Januzzi JL, Pascual-Figal D, Daniels LB. ST2 testing for chronic heart failure therapy monitoring: the International ST2 Consensus Panel. Am J Cardiol 2015;115:70B-75B. [Crossref] [PubMed]
- Watson CJ, Tea I, O'Connell E, et al. Comparison of longitudinal change in sST2 vs BNP to predict major adverse cardiovascular events in asymptomatic patients in the community. J Cell Mol Med 2020;24:6495-9. [Crossref] [PubMed]
- Gaggin HK, Szymonifka J, Bhardwaj A, et al. Head-to-head comparison of serial soluble ST2, growth differentiation factor-15, and highly-sensitive troponin T measurements in patients with chronic heart failure. JACC Heart Fail 2014;2:65-72. [Crossref] [PubMed]
- Somuncu MU, Akgun T, Cakır MO, et al. The Elevated Soluble ST2 Predicts No-Reflow Phenomenon in ST-Elevation Myocardial Infarction Undergoing Primary Percutaneous Coronary Intervention. J Atheroscler Thromb 2019;26:970-8. [Crossref] [PubMed]
- Vílchez JA, Pérez-Cuellar M, Marín F, et al. sST2 levels are associated with all-cause mortality in anticoagulated patients with atrial fibrillation. Eur J Clin Invest 2015;45:899-905. [Crossref] [PubMed]
- Johnpulle RA, Paczesny S, Jung DK, et al. Metabolic Complications Precede Alloreactivity and Are Characterized by Changes in Suppression of Tumorigenicity 2 Signaling. Biol Blood Marrow Transplant 2017;23:529-32. [Crossref] [PubMed]
- Hu X, Zhang H, Song Y, et al. Soluble ST2 is associated with increased carotid intima-media thickness in patients with type 2 diabetes mellitus: A case-control study. Medicine (Baltimore) 2020;99:e18940. [Crossref] [PubMed]
- Lin YH, Zhang RC, Hou LB, et al. Distribution and clinical association of plasma soluble ST2 during the development of type 2 diabetes. Diabetes Res Clin Pract 2016;118:140-5. [Crossref] [PubMed]
- Samuelsson M, Dereke J, Svensson MK, et al. Soluble plasma proteins ST2 and CD163 as early biomarkers of nephropathy in Swedish patients with diabetes, 15-34 years of age: a prospective cohort study. Diabetol Metab Syndr 2017;9:41. [Crossref] [PubMed]
- Caporali A, Meloni M, Miller AM, et al. Soluble ST2 is regulated by p75 neurotrophin receptor and predicts mortality in diabetic patients with critical limb ischemia. Arterioscler Thromb Vasc Biol 2012;32:e149-e160. [Crossref] [PubMed]
- Cardellini M, Rizza S, Casagrande V, et al. Soluble ST2 is a biomarker for cardiovascular mortality related to abnormal glucose metabolism in high-risk subjects. Acta Diabetol 2019;56:273-80. [Crossref] [PubMed]
- Berezin AE. Diabetes mellitus related biomarker: The predictive role of growth-differentiation factor-15. Diabetes Metab Syndr 2016;10:S154-S157. [Crossref] [PubMed]
- Adela R, Banerjee SK. GDF-15 as a Target and Biomarker for Diabetes and Cardiovascular Diseases: A Translational Prospective. J Diabetes Res 2015;2015:490842. [Crossref] [PubMed]
- Desmedt S, Desmedt V, De Vos L, et al. Growth differentiation factor 15: A novel biomarker with high clinical potential. Crit Rev Clin Lab Sci 2019;56:333-50. [Crossref] [PubMed]
- Tsai VWW, Husaini Y, Sainsbury A, et al. The MIC-1/GDF15-GFRAL Pathway in Energy Homeostasis: Implications for Obesity, Cachexia, and Other Associated Diseases. Cell Metab 2018;28:353-68. [Crossref] [PubMed]
- Dong X, Nao J. Association of serum growth differentiation factor 15 level with acute ischemic stroke in a Chinese population. Int J Neurosci 2019;129:1247-55. [Crossref] [PubMed]
- Brenière C, Méloux A, Pédard M, et al. growth differentiation factor-15 (GDF-15) is associated with mortality in ischemic stroke patients treated with acute revascularization therapy. Front Neurol 2019;10:611. [Crossref] [PubMed]
- Shin MY, Kim JM, Kang YE, et al. Association between Growth Differentiation Factor 15 (GDF15) and Cardiovascular Risk in Patients with Newly Diagnosed Type 2 Diabetes Mellitus. J Korean Med Sci 2016;31:1413-8. [Crossref] [PubMed]
- Bao X, Borné Y, Muhammad IF, et al. Growth differentiation factor 15 is positively associated with incidence of diabetes mellitus: the Malmö Diet and Cancer-Cardiovascular Cohort. Diabetologia 2019;62:78-86. [Crossref] [PubMed]
- Gerstein HC, Pare G, Hess S, et al. Growth differentiation factor 15 as a novel biomarker for metformin. Diabetes Care 2017;40:280-3. [Crossref] [PubMed]
- Natali A, Nesti L, Venturi E, et al. Metformin is the key factor in elevated plasma growth differentiation factor-15 levels in type 2 diabetes: a nested, case-control study. Diabetes Obes Metab 2019;21:412-6. [Crossref] [PubMed]
- Kempf T, Guba-Quint A, Torgerson J, et al. Growth differentiation factor 15 predicts future insulin resistance and impaired glucose control in obese nondiabetic individuals: results from the XENDOS trial. Eur J Endocrinol 2012;167:671-8. [Crossref] [PubMed]
- Frimodt-Møller M, von Scholten BJ, Reinhard H, et al. Growth differentiation factor-15 and fibroblast growth factor-23 are associated with mortality in type 2 diabetes - An observational follow-up study. PLoS One 2018;13:e0196634. [Crossref] [PubMed]
- Dominguez-Rodriguez A, Abreu-Gonzalez P, Avanzas P. Usefulness of growth differentiation factor-15 levels to predict diabetic cardiomyopathy in asymptomatic patients with type 2 diabetes mellitus. Am J Cardiol 2014;114:890-4. [Crossref] [PubMed]
- Hellemons ME, Mazagova M, Gansevoort RT, et al. Growth-differentiation factor 15 predicts worsening of albuminuria in patients with type 2 diabetes. Diabetes Care 2012;35:2340-6. [Crossref] [PubMed]
- Scheen AJ. Cardiovascular effects of dipeptidyl peptidase-4 inhibitors: from risk factors to clinical outcomes. Postgrad Med 2013;125:7-20. [Crossref] [PubMed]
- Zelniker TA, Morrow DA, Mosenzon O, et al. Cardiac and Inflammatory Biomarkers Are Associated with Worsening Renal Outcomes in Patients with Type 2 Diabetes Mellitus: Observations from SAVOR-TIMI 53. Clin Chem 2019;65:781-90. [Crossref] [PubMed]
- Scirica BM, Braunwald E, Raz I, et al. Heart failure, saxagliptin, and diabetes mellitus: observations from the SAVOR-TIMI 53 randomized trial. Circulation 2014;130:1579-88. [Crossref] [PubMed]
- Ahrén B. Clinical results of treating type 2 diabetic patients with sitagliptin, vildagliptin or saxagliptin--diabetes control and potential adverse events. Best Pract Res Clin Endocrinol Metab 2009;23:487-98. [Crossref] [PubMed]
- Yu H, Woo VC. Emerging use of combination therapies for the management of type 2 diabetes - focus on saxagliptin and dapagliflozin. Diabetes Metab Syndr Obes 2017;10:317-32. [Crossref] [PubMed]
- Vilsbøll T, Ekholm E, Johnsson E, et al. Efficacy and safety of dapagliflozin plus saxagliptin versus insulin glargine over 52 weeks as add-on to metformin with or without sulphonylurea in patients with type 2 diabetes: A randomized, parallel-design, open-label, Phase 3 trial. Diabetes Obes Metab 2020;22:957-68. [Crossref] [PubMed]
- McMurray JJV, DeMets DL, Inzucchi SE, et al. A trial to evaluate the effect of the sodium-glucose co-transporter 2 inhibitor dapagliflozin on morbidity and mortality in patients with heart failure and reduced left ventricular ejection fraction (DAPA-HF). Eur J Heart Fail 2019;21:665-75. [Crossref] [PubMed]
- Tanaka A, Node K. Exploration of the clinical benefits of sodium glucose co-transporter 2 inhibitors in diabetic patients with concomitant heart failure. Cardiovasc Diabetol 2018;17:74. [Crossref] [PubMed]
- Ghosh RK, Ghosh GC, Gupta M, et al. Sodium Glucose Co-transporter 2 Inhibitors and Heart Failure. Am J Cardiol 2019;124:1790-6. [Crossref] [PubMed]
- Januzzi JL Jr, Butler J, Jarolim P, et al. Effects of Canagliflozin on Cardiovascular Biomarkers in Older Adults With Type 2 Diabetes. J Am Coll Cardiol 2017;70:704-12. [Crossref] [PubMed]
- Nassif ME, Windsor SL, Tang F, et al. Dapagliflozin Effects on Biomarkers, Symptoms, and Functional Status in Patients With Heart Failure With Reduced Ejection Fraction: The DEFINE-HF Trial. Circulation 2019;140:1463-76. [Crossref] [PubMed]
- Cannon CP, McGuire DK, Pratley R, et al. Design and baseline characteristics of the eValuation of ERTugliflozin effIcacy and Safety CardioVascular outcomes trial (VERTIS-CV). Am Heart J 2018;206:11-23. [Crossref] [PubMed]
- Cavender MA, Norhammar A, Birkeland KI, et al. SGLT-2 Inhibitors and Cardiovascular Risk: An Analysis of CVD-REAL. J Am Coll Cardiol 2018;71:2497-506. [Crossref] [PubMed]
- Kim YG, Han SJ, Kim DJ, et al. Association between sodium glucose co-transporter 2 inhibitors and a reduced risk of heart failure in patients with type 2 diabetes mellitus: a real-world nationwide population-based cohort study. Cardiovasc Diabetol 2018;17:91. [Crossref] [PubMed]
- Brito D, Bettencourt P, Carvalho D, et al. Sodium-Glucose Co-transporter 2 Inhibitors in the Failing Heart: a Growing Potential. Cardiovasc Drugs Ther 2020;34:419-36. [Crossref] [PubMed]
- Htike ZZ, Zaccardi F, Papamargaritis D, et al. Efficacy and safety of glucagon-like peptide-1 receptor agonists in type 2 diabetes: A systematic review and mixed-treatment comparison analysis. Diabetes Obes Metab 2017;19:524-36. [Crossref] [PubMed]
- Seferović PM, Petrie MC, Filippatos GS, et al. Type 2 diabetes mellitus and heart failure: a position statement from the Heart Failure Association of the European Society of Cardiology. Eur J Heart Fail 2018;20:853-72. [Crossref] [PubMed]
- Abreu BJ, de Brito Vieira WH. Metalloproteinase Changes in Diabetes. Adv Exp Med Biol 2016;920:185-90. [Crossref] [PubMed]
- Naduk-Kik J, Hrabec E. The role of matrix metalloproteinases in the pathogenesis of diabetes mellitus and progression of diabetes retinopathy. Postepy Hig Med Dosw (Online) 2008;62:442-50. [PubMed]
- Kowluru RA, Zhong Q, Santos JM. Matrix metalloproteinases in diabetic retinopathy: potential role of MMP-9. Expert Opin Investig Drugs 2012;21:797-805. [Crossref] [PubMed]
- Wang X, Li KF, Adams E, et al. Matrix metalloproteinase inhibitors: a review on bioanalytical methods, pharmacokinetics and metabolism. Curr Drug Metab 2011;12:395-410. [Crossref] [PubMed]
- Page-McCaw A, Ewald AJ, Werb Z. Matrix metalloproteinases and the regulation of tissue remodelling. Nat Rev Mol Cell Biol 2007;8:221-33. [Crossref] [PubMed]
- Yiqin Y, Meilin X, Jie X, et al. Aspirin inhibits MMP-2 and MMP-9 expression and activity through PPARalpha/gamma and TIMP-1-mediated mechanisms in cultured mouse celiac macrophages. Inflammation 2009;32:233-41. [Crossref] [PubMed]
- Zhang P, Wu C, Huang XH, et al. Aspirin suppresses TNF-α-induced MMP-9 expression via NF-κB and MAPK signaling pathways in RAW264.7 cells. Exp Ther Med 2017;14:5597-604. [Crossref] [PubMed]
- Das S, Mandal M, Chakraborti T, et al. Isolation of MMP-2 from MMP-2/TIMP-2 complex: characterization of the complex and the free enzyme in pulmonary vascular smooth muscle plasma membrane. Biochim Biophys Acta 2004;1674:158-74. [PubMed]
- Benjamin LE. Glucose, VEGF-A, and diabetic complications. Am J Pathol 2001;158:1181-4. [Crossref] [PubMed]
- Dong Y, Wan G, Yan P, et al. Fabrication of resveratrol coated gold nanoparticles and investigation of their effect on diabetic retinopathy in streptozotocin induced diabetic rats. J Photochem Photobiol B 2019;195:51-7. [Crossref] [PubMed]
- Aiello LP, Wong JS. Role of vascular endothelial growth factor in diabetic vascular complications. Kidney Int Suppl 2000;77:S113-S119. [Crossref] [PubMed]
- Zisa D, Shabbir A, Mastri M, et al. Intramuscular VEGF repairs the failing heart: role of host-derived growth factors and mobilization of progenitor cells. Am J Physiol Regul Integr Comp Physiol 2009;297:R1503-R1515. [Crossref] [PubMed]
- Zisa D, Shabbir A, Mastri M, et al. Intramuscular VEGF activates an SDF1-dependent progenitor cell cascade and an SDF1-independent muscle paracrine cascade for cardiac repair. Am J Physiol Heart Circ Physiol 2011;301:H2422-H2432. [Crossref] [PubMed]
- Huang H, Fu J, Tian H. Effects of quercetin and enalapril on amount of PDGF-B and VEGF-1 in kidney of diabetic rats. Sheng Wu Yi Xue Gong Cheng Xue Za Zhi 2005;22:791-4. [PubMed]
- Park KH, Kim YY, Jo YJ, et al. Healthcare Utilization and Treatment Patterns in Diabetic Macular Edema in Korea: a Retrospective Chart Review. J Korean Med Sci 2019;34:e118. [Crossref] [PubMed]
- Li G, Zou X, Zhu Y, et al. Expression and Influence of Matrix Metalloproteinase-9/Tissue Inhibitor of Metalloproteinase-1 and Vascular Endothelial Growth Factor in Diabetic Foot Ulcers. Int J Low Extrem Wounds 2017;16:6-13. [Crossref] [PubMed]
- Ishizaki E, Takai S, Ueki M, et al. Correlation between angiotensin-converting enzyme, vascular endothelial growth factor, and matrix metalloproteinase-9 in the vitreous of eyes with diabetic retinopathy. Am J Ophthalmol 2006;141:129-34. [Crossref] [PubMed]
- Ravichandran S, Srivastav S, Kamble PH, et al. VEGF-A and cardiac autonomic function in newly diagnosed type 2 diabetes mellitus: A cross-sectional study at a tertiary care center. J Family Med Prim Care 2019;8:3185-90. [Crossref] [PubMed]
- Rasmussen HS, Rasmussen CS, Macko J. VEGF gene therapy for coronary artery disease and peripheral vascular disease. Cardiovasc Radiat Med 2002;3:114-7. [Crossref] [PubMed]
- Barasch E, Gottdiener JS, Aurigemma G, et al. The relationship between serum markers of collagen turnover and cardiovascular outcome in the elderly: the Cardiovascular Health Study. Circ Heart Fail 2011;4:733-9. [Crossref] [PubMed]
- Berezin AE, Kremzer AA. Circulating osteopontin as a marker of early coronary vascular calcification in type two diabetes mellitus patients with known asymptomatic coronary artery disease. Atherosclerosis 2013;229:475-81. [Crossref] [PubMed]
- Tousoulis D, Siasos G, Maniatis K, et al. Serum osteoprotegerin and osteopontin levels are associated with arterial stiffness and the presence and severity of coronary artery disease. Int J Cardiol 2013;167:1924-8. [Crossref] [PubMed]
- Kadoglou NP, Gerasimidis T, Golemati S, et al. The relationship between serum levels of vascular calcification inhibitors and carotid plaque vulnerability. J Vasc Surg 2008;47:55-62. [Crossref] [PubMed]
- Kadoglou NP, Gerasimidis T, Kapelouzou A, et al. Beneficial changes of serum calcification markers and contralateral carotid plaques echogenicity after combined carotid artery stenting plus intensive lipid-lowering therapy in patients with bilateral carotid stenosis. Eur J Vasc Endovasc Surg 2010;39:258-65. [Crossref] [PubMed]
- Nagao K, Inada T, Tamura A, et al. Circulating markers of collagen types I, III, and IV in patients with dilated cardiomyopathy: relationships with myocardial collagen expression. ESC Heart Fail 2018;5:1044-51. [Crossref] [PubMed]
- Hygum K, Starup-Linde J, Harsløf T, et al. MECHANISMS IN ENDOCRINOLOGY: Diabetes mellitus, a state of low bone turnover - a systematic review and meta-analysis. Eur J Endocrinol 2017;176:R137-R157. [Crossref] [PubMed]
- Hussein RM. Biochemical relationships between bone turnover markers and blood glucose in patients with type 2 diabetes mellitus. Diabetes Metab Syndr 2017;11:S369-S372. [Crossref] [PubMed]
- Starup-Linde J, Vestergaard P. Biochemical bone turnover markers in diabetes mellitus - A systematic review. Bone 2016;82:69-78. [Crossref] [PubMed]
- Futrakul N, Futrakul P. Biomarker for early renal microvascular and diabetic kidney diseases. Ren Fail 2017;39:505-11. [Crossref] [PubMed]
- Liu JH, Chen Y, Zhen Z, et al. Relationship of biomarkers of extracellular matrix with myocardial function in Type 2 diabetes mellitus. Biomark Med 2017;11:569-78. [Crossref] [PubMed]
- Purnamasari D, Puspitasari MD, Setiyohadi B, et al. Low bone turnover in premenopausal women with type 2 diabetes mellitus as an early process of diabetes-associated bone alterations: a cross-sectional study. BMC Endocr Disord 2017;17:72. [Crossref] [PubMed]
- Asahara T, Murohara T, Sullivan A, et al. Isolation of putative progenitor endothelial cells for angiogenesis. Science 1997;275:964-7. [Crossref] [PubMed]
- Pyšná A, Bém R, Němcová A, et al. Endothelial progenitor cells biology in diabetes mellitus and peripheral arterial disease and their therapeutic potential. Stem Cell Rev Rep 2019;15:157-65. [Crossref] [PubMed]
- Berezin AE. Endothelial progenitor cells dysfunction and impaired tissue reparation: The missed link in diabetes mellitus development. Diabetes Metab Syndr 2017;11:215-20. [Crossref] [PubMed]
- Menegazzo L, Albiero M, Avogaro A, Fadini GP. Endothelial progenitor cells in diabetes mellitus. Biofactors 2012;38:194-202. [Crossref] [PubMed]
- Zahran AM, Mohamed IL, El Asheer OM, et al. Circulating Endothelial Cells, Circulating Endothelial Progenitor Cells, and Circulating Microparticles in Type 1 Diabetes Mellitus. Clin Appl Thromb Hemost 2019;25:1076029618825311. [Crossref] [PubMed]
- Shao Y, Chen J, Dong LJ, et al. A Protective Effect of PPARα in Endothelial Progenitor Cells Through Regulating Metabolism. Diabetes 2019;68:2131-42. [Crossref] [PubMed]
- Berezin A, Zulli A, Kerrigan S, et al. Predictive role of circulating endothelial-derived microparticles in cardiovascular diseases. Clin Biochem 2015;48:562-8. [Crossref] [PubMed]
- Wils J, Favre J, Bellien J. Modulating putative endothelial progenitor cells for the treatment of endothelial dysfunction and cardiovascular complications in diabetes. Pharmacol Ther 2017;170:98-115. [Crossref] [PubMed]
- Egan CG, Fondelli C, Pierantozzi E, et al. Putative endothelial progenitor cells predict long-term mortality in type-2 diabetes. Endocrine 2018;62:263-6. [Crossref] [PubMed]
- Hassanpour M, Rezabakhsh A, Rahbarghazi R, et al. Functional convergence of Akt protein with VEGFR-1 in human endothelial progenitor cells exposed to sera from patient with type 2 diabetes mellitus. Microvasc Res 2017;114:101-13. [Crossref] [PubMed]
- Falay M, Aktas S. Endothelial Progenitor Cells (EPC) Count by Multicolor Flow Cytometry in Healthy Individuals and Diabetes Mellitus (DM) Patients. Clin Lab 2016;62:2161-6. [Crossref] [PubMed]
- Martínez MC, Andriantsitohaina R. Extracellular vesicles in metabolic syndrome. Circ Res 2017;120:1674-86. [Crossref] [PubMed]
- Wu ZH, Ji CL, Li H, et al. Membrane microparticles and diseases. Eur Rev Med Pharmacol Sci 2013;17:2420-7. [PubMed]
- Berezin AE, Kremzer AA, Martovitskaya YV, et al. Pattern of endothelial progenitor cells and apoptotic endothelial cell-derived microparticles in chronic heart failure patients with preserved and reduced left ventricular ejection fraction. EBioMedicine 2016;4:86-94. [Crossref] [PubMed]
- Chen F, Zhang H, Wang Z, et al. Adipose-Derived Stem Cell-Derived Exosomes Ameliorate Erectile Dysfunction in a Rat Model of Type 2 Diabetes. J Sex Med 2017;14:1084-94. [Crossref] [PubMed]
- Zuwala-Jagiello J, Pazgan-Simon M, Murawska-Cialowicz E, et al. Influence of Diabetes on Circulating Apoptotic Microparticles in Patients with Chronic Hepatitis C. In Vivo 2017;31:1027-34. [PubMed]
- Jansen F, Yang X, Hoelscher M, et al. Endothelial microparticle-mediated transfer of MicroRNA-126 promotes vascular endothelial cell repair via SPRED1 and is abrogated in glucose-damaged endothelial microparticles. Circulation 2013;128:2026-38. [Crossref] [PubMed]
- Giannella A, Radu CM, Franco L, et al. Circulating levels and characterization of microparticles in patients with different degrees of glucose tolerance. Cardiovasc Diabetol 2017;16:118. [Crossref] [PubMed]
- Li S, Wei J, Zhang C, et al. Cell-Derived Microparticles in Patients with Type 2 Diabetes Mellitus: a Systematic Review and Meta-Analysis. Cell Physiol Biochem 2016;39:2439-50. [Crossref] [PubMed]
- Wang B, Cai W, Zhang Z, et al. Circulating microparticles in patients after ischemic stroke: a systematic review and meta-analysis. Rev Neurosci 2018; [Epub ahead of print]. [Crossref] [PubMed]
- Wang B, Li T, Han X, et al. The level of circulating microparticles in patients with coronary heart disease: a systematic review and meta-analysis. J Cardiovasc Transl Res 2020;13:702-12. [Crossref] [PubMed]
- Nichols S, Nation F, Goodman T, et al. CARE CR-Cardiovascular and cardiorespiratory Adaptations to Routine Exercise-based Cardiac Rehabilitation: a study protocol for a community-based controlled study with criterion methods. BMJ Open 2018;8:e019216. [Crossref] [PubMed]
- Dias KA, Ingul CB, Tjønna AE, et al. Effect of High-Intensity Interval Training on Fitness, Fat Mass and Cardiometabolic Biomarkers in Children with Obesity: A Randomised Controlled Trial. Sports Med 2018;48:733-46. [Crossref] [PubMed]
- Phillips SA, Kuguimoto Andaku D, Gonçalves Mendes R, et al. Exploring vascular function biomarkers: implications for rehabilitation. Braz J Cardiovasc Surg 2017;32:125-35. [Crossref] [PubMed]
- Van Craenenbroeck EM, Frederix G, Pattyn N, et al. Effects of aerobic interval training and continuous training on cellular markers of endothelial integrity in coronary artery disease: a SAINTEX-CAD substudy. Am J Physiol Heart Circ Physiol 2015;309:H1876-H1882. [Crossref] [PubMed]
- Stewart RAH, Held C, Hadziosmanovic N, et al. Physical Activity and Mortality in Patients With Stable Coronary Heart Disease. J Am Coll Cardiol 2017;70:1689-700. [Crossref] [PubMed]
- Piepoli MF, Hoes AW, Agewall S, et al. 2016 European Guidelines on cardiovascular disease prevention in clinical practice: The Sixth Joint Task Force of the European Society of Cardiology and Other Societies on Cardiovascular Disease Prevention in Clinical Practice (constituted by representatives of 10 societies and by invited experts) Developed with the special contribution of the European Association for Cardiovascular Prevention & Rehabilitation (EACPR). Eur Heart J 2016;37:2315-81. [Crossref] [PubMed]
- Gokce N, Keaney JF Jr, Hunter LM, et al. Risk stratification for postoperative cardiovascular events via noninvasive assessment of endothelial function: a prospective study. Circulation 2002;105:1567-72. [Crossref] [PubMed]
Cite this article as: Berezin AE, Berezin AA. Challenging and opportunities in clinical implementation of circulating cardiac biomarkers in diabetes mellitus. AME Med J 2021;6:18.