Frontiers in post-radiation urologic reconstruction; robotic surgery and near-infrared fluorescence imaging
Introduction
Radiation therapy is an important treatment option for management of pelvic organ malignancies including urologic, gynecologic, and gastrointestinal cancers (1). Radiation toxicity results from DNA and cellular damage of healthy tissue in the radiation field and can ultimately lead to tissue necrosis or fibrosis. Radiation therapy of abdominal and pelvic malignancies can cause delayed adverse functional and anatomical effects that involve portions of the urinary tract, such as the ureters, bladder, and posterior urethra. These adverse effects are believed to result from damage to urinary tract epithelium and microvasculature (2,3). Ureteral stricture, contracted bladder, rectourethral fistula, bladder neck contracture, and urethral stricture disease are among the long-term toxicities of pelvic radiation and usually require surgical intervention (4-7). Classically, open surgery has been considered the preferred approach for management of these conditions, potentially due to easier tissue handling and manipulation compared to minimally invasive techniques like laparoscopy. However, open surgery, especially in a radiated field, can be associated with higher morbidity than minimally invasive surgery with regard to wound complications, extended recovery and lower success with additional interventions and revisions needed (8). Newer technologies, such as robotic surgery, were introduced in the early 2000s. This technology, among other advancements, has continued to be developed and implemented as a feasible alternative to classic surgical techniques and approaches (9). In this review article, we will discuss the role of recent advancements in reconstructive surgery for urologic pathology following pelvic radiation. A PubMed search for all published articles and book chapters was performed using the following key words: post-radiation, urologic reconstruction, robotic surgery, immunofluorescence imaging, indocyanine green, ureteroplasty, bladder reconstruction, bladder neck reconstruction, and urethral reconstruction.
Considerations for reconstruction of radiated tissue
The objective of successful urological reconstruction is to restore healthy, patent, and functional urinary tract structures to allow normal storage and passage of urine through the urinary tract. This objective is achieved through a variety of techniques including excision of fibrotic, unhealthy tissue and either mobilization of healthy urinary tract tissue or incorporation of extra-urinary tissue flaps (e.g., intestinal, muscle or omental flaps) or grafts (e.g., oral mucosa or rectal mucosa) to bridge the gap created by the abnormal tissue. The main challenge in radiated tissue reconstruction is the extensive tissue ischemia and fibrosis induced by radiation. Meticulous dissection and fine tissue handling are crucial to allow preservation of blood supply to the tissue that is being used in the reconstruction to prevent short term complications such as lack of healing, disruption of the anastomosis, and long-term complications such as recurrence of fibrosis and obstruction. Also, it is important to consider the effect of previous radiation on surrounding organs such as bowel, which may limit the availability of ileal or appendiceal flaps or other local tissue for reconstruction. Another consideration during post-radiation reconstruction is to provide a healthy vascular bed to support a potential graft. This can be challenging in a radiated field as application of graft on ischemic radiated bed can decrease the chances of graft survival. Harvesting a nearby healthy flap to be used as a bed for the graft can be considered if there are concerns regarding the viability of local tissue (8,10).
Robotic surgery in post-radiation urologic reconstruction
In 2000, Intuitive’s da Vinci™ surgical robotic system was approved by the Federal Drug Administration (FDA) for laparoscopic surgery (9). Robotic surgery was then incorporated into many urological procedures and has subsequently become an essential part of current urologic practice. Although robotic technology is widely used in urologic oncology procedures, it has also been adopted early in the field of reconstructive urology. In 2005, the use of the robotic platform was reported in pyeloplasties in 50 adult patients (11). After these techniques were established for robotic pyeloplasty, they were then applied to other procedures for upper urinary tract reconstruction (11,12). The unpredictable extent of tissue fibrosis following radiation and the lack of viable tissue make reconstructive urologic procedures quite challenging and may explain the lag in implementation of the robotic approach in post-radiation reconstruction (8). However, robotic technology provides many advantages that may improve outcomes in this group of patients. In a series of open post-radiation urologic reconstructive procedures, the rate of post-operative morbidities was reported to be as high as 54% (8). The robotic approach has been reported to be associated with decreased early post-operative morbidities in other major urological procedures, which can be applied to post-radiation reconstruction to improve outcomes and decrease morbidities (13,14).
Robotic technology provides a 3D image with 10 to 15 folds magnification that enhances visualization of anatomical details, which is a particularly important advantage in radiated fields with dense scar reaction. In addition, small robotic instruments allow for better visualization and tissue manipulation in deep and hard to access areas known to be challenging using an open approach, such as bladder neck reconstruction. The wide degree of movement of articulated instruments and the dexterity provided aids in precise tissue dissection and easier suturing in narrow deep spaces, which is pivotal in reconstructive procedures (15).
Near-infrared fluorescence (NIRF) imaging “NIRF” technology
NIRF is one of many modalities that is being used for fluorescence image-guided surgery. This technology has been used in different settings, including open, microscopic, and minimally invasive approaches. It aims to enhance the surgeon’s ability to identify anatomical details that are difficult to be visualized under white light (16-18). This technology has become even more convenient for use in robotic surgery with the TilePro™ feature, which allows the surgeon to easily toggle between white light 3D and NIRF on the robotic console. NIRF was integrated with the da Vinci™ robotic system in 2009 under the name “FireFly™” to provide fluorescence imaging intraoperatively (18,19). NIRF imaging requires a NIR light source, collection optics, filter, and camera. Together with administration of a NIR contrast material that can be administered either intravenously or intraluminally, a fluorescence image is then incorporated with the white light image of the surgical field and displayed on the monitor (17,18). NIR light is invisible to the naked eye and requires a specialized camera for the light to be displayed on a monitor or the surgeon console. NIR light has a higher tissue penetration than white light and can penetrate up to 5–8 mm through tissue, which helps to better identify anatomical details of deeper structures (16,18,20).
Indocyanine green (ICG)
Indocyanine green is a water-soluble dye that can be used as a fluorescence contrast in combination with NIR imaging (18). It was FDA approved for medical use in 1956 for evaluation of cardiac output, and later approved for hepatic and retinal angiography (17,18). ICG has been proven to be safe and effective and is now widely used in many different clinical applications. However, there are some side effects that need to be considered: in patients who are allergic to iodine, it can cause an allergic or anaphylactic reaction and can cause fatal arrhythmias if used 6 hours after preparation with saline. Therefore, ICG must be prepared immediately before administration and discarded after 6 hours (17,18).
The combination of robotic NIRF technology and ICG was readily adopted by robotic urologic surgeons (17,18). When injected intravenously, ICG conjugates with plasma albumin and has a short half-life in the blood vessels (2.5–3 min), making it excellent for angiography and evaluation of tissue perfusion. By instilling ICG directly into structures such as the bladder or ureter, this technology can also aid in identification in an operative field with altered anatomy and fibrosis, such as previously radiated tissue (17,18,21) (Figure 1). The ability to evaluate tissue perfusion and to aid in structure identification intraoperatively are the main reasons that ICG/NIRF is becoming increasingly popular among reconstructive urologists in robotic surgery.
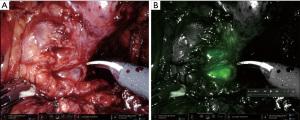
Intraluminal white light detection with NIRF
In their video article describing the technique for robotic bladder neck reconstruction, Granieri et al. described the use of intraluminal white light detection using the FireFly™ robotic feature with the da Vinci™ platform (22). By advancing a cystoscope via the urethra up to the level of urethral stenosis, they were able to utilize FireFly™ vision to identify the urethral lumen and isolate the proximal end under fluorescence guidance, as even the white light from the scope is able to be detected using NIRF technology. This technique does not require the use of ICG or any other NIR contrast (22). Similarly, this same technique can be used to identify the edges of the bladder in a patient with dense adhesions and scar as well as the ureteral lumen and stricture site using the light emitted from a ureteroscope (Figure 2).
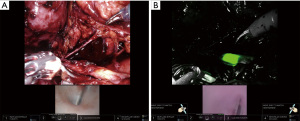
Applications of new technology in post-radiation urologic reconstruction
In this section, we will highlight the application of aforementioned technologies in the field of post-radiation urologic reconstruction or in similar situations.
Ureteral reconstruction
With the growing incorporation of robotic surgery in many reconstructive urologic procedures, efforts have been made to mimic the techniques for open ureteral reconstruction with the use of robotic technology (23,24). The Collaborative of Reconstructive Robotic Ureteral Surgery (CORRUS) reported the largest series of robotic reconstruction following radiation in 32 patients and 35 ureteral units. They illustrated available options and techniques that can be employed for challenging post radiation cases. They reported a success rate of 88.2% in this group of patients at 13 months follow-up. It is crucial for the reconstructive surgeon to be familiar with different strategies and techniques, as often the intraoperative findings can be different from preoperative imaging and evaluation. Therefore, having a variety of tools and techniques available can maximize the chance of success. Five major techniques were described: dismembered end-to-end and non-dismembered side-to-side ureteral reimplantation, augmentation ureteroplasty using buccal mucosal and appendiceal flaps, and ureteral segment replacement using ileal interposition. Psoas hitch and Boari flap are adjunct techniques that can be employed to achieve tension free anastomosis when indicated (10).
NIRF imaging is particularly helpful in ureteral reconstruction. It can be used for ureteral identification and visualization with better localization of the strictured ureteral segment via intraluminal injection. ICG can be instilled through a preplaced nephrostomy tube to delineate the proximal ureter (Figure 1) and through a ureteral catheter placed from the bladder to outline the distal ureter as described by Lee et al. (21). Alternatively, ICG can be injected intravenously to evaluate tissue perfusion. A poorly perfused ureteral segment, identified by lack of fluorescent color, maybe excised or trimmed until healthy vascularized tissue is reached prior to starting the anastomosis (18,25). The combination of NIRF technology with intraluminal white light, delivered endoscopically via cystoscope in lower urinary tract reconstruction, can also applied to ureteral reconstruction by using a ureteroscope to identify the level of ureteral stricture and using NIRF imaging to locate the tip of the ureteroscope (22) (Figure 2).
Bladder reconstruction
Bladder contracture and dysfunction are side effects of pelvic radiation that can lead to serious medical morbidity including renal function deterioration, persistent urinary tract infections, lower urinary tract symptoms, or persistent hemorrhagic cystitis (8). In such cases, surgical intervention may be indicated to achieve preservation of renal function and improve quality of life (8). The decision regarding options for reconstruction is usually made on a case-by-case basis due to the complex nature of this pathology. Treatment plans should be made after a thorough and extensive evaluation, taking into account renal function, upper tract anatomy, bladder function and capacity, identification of associated anatomic urinary tract pathology such as fistulae or strictures, and previous abdominal surgeries that could limit options for reconstruction (23). Options for reconstruction in the post-radiation contracted bladder include augmentation ileocystoplasty with or without a catheterizable stoma versus cystectomy with urinary diversion (8). Robotic augmentation cystoplasty in adults has been reported in case reports with no larger studies available. However, these reports illustrate the feasibility of incorporating the robotic approach into a complex procedure (26-28). Urinary diversion is a valid option if the bladder is unsalvageable due to extensive pathology (29). Robotic intracorporeal urinary diversion is currently gaining more popularity with early data suggesting some benefits over the extracorporeal technique, including smaller incisions, decreased fluid loss from exposure of the abdominal contents, decrease postoperative gastrointestinal complications, and decreased manipulation of tissue (30-32). This approach can also be utilized for patients with post-radiation contracted bladder in need of urinary diversion.
Urinary fistulae, including uro-gynecological, uro-cutaneous, and uro-enteric fistulae, can be long-term sequalae of pelvic radiation (8). The use of the robotic approach has been reported in management of vesicovaginal and vesico-rectal fistulae and show the feasibility of robotic surgery in these situations. The benefits and success rates of the robotic assisted radiated fistula repair and closure are considered advanced techniques secondary to rarity and the heterogenic nature of these cases (33-37). A successful repair of a radiated fistula lies in the ability to place a robust interposition flap between the two tissue planes. Whether this is approached robotically or perineally depends on the location of the fistula and access to a well vascularized flap.
Bladder neck reconstruction
Bladder neck contracture or stenosis can result from many treatment modalities for prostate cancer: prostatectomy, radiation therapy, or the combination of both (38). Robotic surgery recently has become part of the treatment algorithm for this pathology. Few reports in the literature describe the robotic approach for bladder neck reconstruction. Although bladder neck reconstruction after transurethral resection prostate resection or bladder outlet reduction surgery is relatively straight forward, the radiated post-radical prostatectomy bladder neck reconstruction is much more challenging. The robotic approach in these cases provides superior visualization, tissue manipulation and precise suturing in the retropubic deep pelvis space. The use of NIRF imaging in combination with intraluminal endoscopic white light helps identify healthy urethral anatomy, accurate scar excision, and precise dissection (22). The TilePro™ feature of the robotic system allows for simultaneous display of images from the robotic camera and cystoscope within the surgeon console during cases, providing side-by-side information regarding the patient’s anatomy (39,40). The addition of NIRF imaging to detect intraluminal white light with direct intraluminal visualization using the TilePro™ feature facilitates identification of abnormal anatomy for scar excision and facilitates reconstruction of the posterior urethra and bladder neck, especially if the lumen is completely obliterated (39,40).
Posterior urethral reconstruction
Reconstruction of the posterior urethra and proximal bulbar urethra is classically done via an open perineal approach. This procedure becomes more challenging when the injured urethra is located high behind the pubic symphysis. A posterior urethroplasty can become even more challenging following radiation where the tissue is fixed and fibrotic as many of the natural planes are obliterated. The scar tissue must be dissected and excised carefully to ensure adequate mobilization of the remaining urethra for a tension free anastomosis (41). Robotic technology can be used to facilitate precise proximal suture placement. In this technique, use of the robotic system to place proximal sutures offers enhanced visualization and superior magnification in the narrow and deep space where suturing is to occur (40,42). The surgeon benefits from improved ergonomics with improved identification of the healthy proximal and distal mucosal edges while avoiding the extreme neck and back positional torsion which often occurs when performing the procedure. This application of robotic technology in post radiation posterior urethroplasty may contribute to improved outcomes in this challenging disease process (41). The role of NIRF/ICG in posterior urethral reconstruction has not yet been studied in the literature. Future studies are needed to evaluate the incorporation of this technology in such cases.
Other applications
The technologies and techniques used in post-radiation reconstruction can also be readily applied to many aspects of urological reconstruction, particularly in other instances with poor tissue quality and severe fibrosis such as patients who have had numerous infections, significant urinary leaks, prior chemotherapy, or previous surgery. As demonstrated by this recent publication on robotic assisted reconstruction of the kidney transplant ureter, prior complex open surgery can be successfully managed using a robotic assisted technique. The authors describe the benefits of the robotic approach with the use of with NIRF and TilePro™ for successful ureteral reimplantation in post kidney transplant ureteral strictures (39).
Future directions
Recently, new technological advancements in the field of minimally invasive and robotic surgery have been introduced and applied to many urologic surgeries. This includes the single-port (SP) platform that was FDA approved in 2018 (43). After it was commercially available, the applications of the new platform have been reported in many urological procedures including reconstructive surgeries (44,45). The double-jointed articulation of the robotic camera and instruments provides better accessibility to deep narrow anatomy with less chance of instrument clashing (43,45). The role of the SP robotic platform in post-radiation reconstruction is yet to be explored and evaluated. Another technology that is currently under investigation is intraluminal trans-endoscopic robotic surgery which may be a new arena for super minimally invasive surgery. The potentials of this technology may become one of the main future focuses of reconstructive urology research (46,47).
Conclusions
Post-radiation urologic reconstructive procedures can be challenging and complex due to extensive tissue ischemia and fibrosis. Recent advances in robotic assisted surgery such as NIRF/ICG technology, improved visualization and access, precise suturing are valuable assets in improving the operative care and recovery in this group of patients.
Acknowledgments
Funding: None.
Footnote
Provenance and Peer Review: This article was commissioned by the Guest Editor (Lucas Wiegand) for the series “Radiation Urologic Reconstruction” published in AME Medical Journal. The article has undergone external peer review.
Peer Review File: Available at https://amj.amegroups.com/article/view/10.21037/amj-21-3/prf
Conflicts of Interest: All authors have completed the ICMJE uniform disclosure form (available at https://amj.amegroups.com/article/view/10.21037/amj-21-3/coif). The series “Radiation Urologic Reconstruction” was commissioned by the editorial office without any funding or sponsorship. The authors have no other conflicts of interest to declare.
Ethical Statement: The authors are accountable for all aspects of the work in ensuring that questions related to the accuracy or integrity of any part of the work are appropriately investigated and resolved.
Open Access Statement: This is an Open Access article distributed in accordance with the Creative Commons Attribution-NonCommercial-NoDerivs 4.0 International License (CC BY-NC-ND 4.0), which permits the non-commercial replication and distribution of the article with the strict proviso that no changes or edits are made and the original work is properly cited (including links to both the formal publication through the relevant DOI and the license). See: https://creativecommons.org/licenses/by-nc-nd/4.0/.
References
- Siegel RL, Miller KD, Jemal A. Cancer statistics, 2015. CA Cancer J Clin 2015;65:5-29. [Crossref] [PubMed]
- Hall EJ, Astor M, Bedford J, et al. Basic radiobiology. Am J Clin Oncol 1988;11:220-52. [Crossref] [PubMed]
- Turina M, Mulhall AM, Mahid SS, et al. Frequency and surgical management of chronic complications related to pelvic radiation. Arch Surg 2008;143:46-52; discussion 52. [Crossref] [PubMed]
- Wang W, Hou X, Yan J, et al. Outcome and toxicity of radical radiotherapy or concurrent Chemoradiotherapy for elderly cervical cancer women. BMC Cancer 2017;17:510. [Crossref] [PubMed]
- Kim S, Moore DF, Shih W, et al. Severe genitourinary toxicity following radiation therapy for prostate cancer--how long does it last? J Urol 2013;189:116-21. [Crossref] [PubMed]
- McIntyre JF, Eifel PJ, Levenback C, et al. Ureteral stricture as a late complication of radiotherapy for stage IB carcinoma of the uterine cervix. Cancer 1995;75:836-43. [Crossref] [PubMed]
- Zoubek J, McGuire EJ, Noll F, et al. The late occurrence of urinary tract damage in patients successfully treated by radiotherapy for cervical carcinoma. J Urol 1989;141:1347-9. [Crossref] [PubMed]
- Toia B, Seth J, Ecclestone H, et al. Outcomes of reconstructive urinary tract surgery after pelvic radiotherapy. Scand J Urol 2019;53:156-60. [Crossref] [PubMed]
- Pugin F, Bucher P, Morel P. History of robotic surgery: from AESOP® and ZEUS® to da Vinci®. J Visc Surg 2011;148:e3-8. [Crossref] [PubMed]
- Asghar AM, Lee Z, Lee RA, et al. Robotic Ureteral Reconstruction in Patients with Radiation-Induced Ureteral Strictures: Experience from the Collaborative of Reconstructive Robotic Ureteral Surgery. J Endourol 2021;35:144-50. [Crossref] [PubMed]
- Patel V. Robotic-assisted laparoscopic dismembered pyeloplasty. Urology 2005;66:45-9. [Crossref] [PubMed]
- Mufarrij PW, Shah OD, Berger AD, et al. Robotic reconstruction of the upper urinary tract. J Urol 2007;178:2002-5. [Crossref] [PubMed]
- Flamiatos JF, Chen Y, Lambert WE, et al. Open versus robot-assisted radical cystectomy: 30-day perioperative comparison and predictors for cost-to-patient, complication, and readmission. J Robot Surg 2019;13:129-40. [Crossref] [PubMed]
- Khalil MI, Tourchi A, Langford BT, et al. Early Postoperative Morbidity of Robotic Versus Open Radical Cystectomy in Obese Patients. J Endourol 2020;34:461-8. [Crossref] [PubMed]
- Thiel DD, Winfield HN. Robotics in urology: past, present, and future. J Endourol 2008;22:825-30. [Crossref] [PubMed]
- Vahrmeijer AL, Hutteman M, van der Vorst JR, et al. Image-guided cancer surgery using near-infrared fluorescence. Nat Rev Clin Oncol 2013;10:507-18. [Crossref] [PubMed]
- Marshall MV, Rasmussen JC, Tan IC, et al. Near-Infrared Fluorescence Imaging in Humans with Indocyanine Green: A Review and Update. Open Surg Oncol J 2010;2:12-25. [Crossref] [PubMed]
- Liao JC, Su LM. Advances in Image-Guided Urologic Surgery. New York: Springer, 2015.
- Tobis S, Knopf J, Silvers C, et al. Near infrared fluorescence imaging with robotic assisted laparoscopic partial nephrectomy: initial clinical experience for renal cortical tumors. J Urol 2011;186:47-52. [Crossref] [PubMed]
- Alander JT, Kaartinen I, Laakso A, et al. A review of indocyanine green fluorescent imaging in surgery. Int J Biomed Imaging 2012;2012:940585. [Crossref] [PubMed]
- Lee Z, Simhan J, Parker DC, et al. Novel use of indocyanine green for intraoperative, real-time localization of ureteral stenosis during robot-assisted ureteroureterostomy. Urology 2013;82:729-33. [Crossref] [PubMed]
- Granieri MA, Weinberg AC, Sun JY, et al. Robotic Y-V Plasty for Recalcitrant Bladder Neck Contracture. Urology 2018;117:163-5. [Crossref] [PubMed]
- Patel VR, Ramalingam M. Operative Atlas of Laparoscopic and Robotic Reconstructive Urology. Second edition. Cham, Switzerland: Springer, 2017.
- Lee Z, Sehgal S, Llukani E, et al. Single-surgeon experience with robot-assisted ureteroneocystostomy for distal ureteral pathologies in adults. Korean J Urol 2013;54:516-21. [Crossref] [PubMed]
- Bjurlin MA, Gan M, McClintock TR, et al. Near-infrared fluorescence imaging: emerging applications in robotic upper urinary tract surgery. Eur Urol 2014;65:793-801. [Crossref] [PubMed]
- Kang IS, Lee JW, Seo IY. Robot-assisted laparoscopic augmentation ileocystoplasty: a case report. Int Neurourol J 2010;14:61-4. [Crossref] [PubMed]
- Dogra PN, Regmi SK, Singh P, et al. Robot-assisted laparoscopic augmentation ileocystoplasty in a tubercular bladder. Urol Ann 2014;6:152-5. [Crossref] [PubMed]
- Caputo PA, Ramirez D, Maurice M, et al. Robotic Assisted Laparoscopic Augmentation Ileocystoplasty. Int Braz J Urol 2017;43:994. [Crossref] [PubMed]
- Faris SF, Milam DF, Dmochowski RR, et al. Urinary diversions after radiation for prostate cancer: indications and treatment. Urology 2014;84:702-6. [Crossref] [PubMed]
- Dal Moro F, Haber GP, Wiklund P, et al. Robotic intracorporeal urinary diversion: practical review of current surgical techniques. Minerva Urol Nefrol 2017;69:14-25. [PubMed]
- Hussein AA, May PR, Jing Z, et al. Outcomes of Intracorporeal Urinary Diversion after Robot-Assisted Radical Cystectomy: Results from the International Robotic Cystectomy Consortium. J Urol 2018;199:1302-11. [Crossref] [PubMed]
- Cacciamani GE, Rajarubendra N, Artibani W, et al. Robotic intracorporeal urinary diversion: state of the art. Curr Opin Urol 2019;29:293-300. [Crossref] [PubMed]
- Jairath A. Robotic repair of vesicovaginal fistula - initial experience. Int Braz J Urol 2016;42:168-9. [Crossref] [PubMed]
- Martini A, Dattolo E, Frizzi J, et al. Robotic vesico-vaginal fistula repair with no omental flap interposition. Int Urogynecol J 2016;27:1277-8. [Crossref] [PubMed]
- Bora GS, Singh S, Mavuduru RS, et al. Robot-assisted vesicovaginal fistula repair: a safe and feasible technique. Int Urogynecol J 2017;28:957-62. [Crossref] [PubMed]
- Sotelo R, Medina LG, Husain FZ, et al. Robotic-assisted laparoscopic repair of rectovesical fistula after Hartmann's reversal procedure. J Robot Surg 2019;13:339-43. [Crossref] [PubMed]
- Oderda M, Bonet X, Campobasso D, et al. Robotic rectovesical fistula repair: a successful approach. J Laparoendosc Adv Surg Tech A 2014;24:567-70. [Crossref] [PubMed]
- Browne BM, Vanni AJ. Management of Urethral Stricture and Bladder Neck Contracture Following Primary and Salvage Treatment of Prostate Cancer. Curr Urol Rep 2017;18:76. [Crossref] [PubMed]
- Kim S, Fuller TW, Buckley JC. Robotic Surgery for the Reconstruction of Transplant Ureteral Strictures. Urology 2020;144:208-13. [Crossref] [PubMed]
- Kim S, Buckley JC. Robotic Lower Urinary Tract Reconstruction. Urol Clin North Am 2021;48:103-12. [Crossref] [PubMed]
- Fuchs JS, Hofer MD, Sheth KR, et al. Improving Outcomes of Bulbomembranous Urethroplasty for Radiation-induced Urethral Strictures in Post-Urolume Era. Urology 2017;99:240-5. [Crossref] [PubMed]
- Unterberg SH, Patel SH, Fuller TW, et al. Robotic-assisted Proximal Perineal Urethroplasty: Improving Visualization and Ergonomics. Urology 2019;125:230-3. [Crossref] [PubMed]
- Bertolo R, Garisto J, Gettman M, et al. Novel System for Robotic Single-port Surgery: Feasibility and State of the Art in Urology. Eur Urol Focus 2018;4:669-73. [Crossref] [PubMed]
- Garbens A, Morgan T, Cadeddu JA. Single Port Robotic Surgery in Urology. Curr Urol Rep 2021;22:22. [Crossref] [PubMed]
- Garisto J, Bertolo R, Reese SW, et al. Minimizing minimally invasive surgery: Current status of the single-port robotic surgery in Urology. Actas Urol Esp 2021;45:345-52. (Engl Ed). [Crossref] [PubMed]
- Hendrick RJ, Mitchell CR, Herrell SD, et al. Hand-held transendoscopic robotic manipulators: A transurethral laser prostate surgery case study. Int J Rob Res 2015;34:1559-72. [Crossref] [PubMed]
- Amanov E, Ropella DS, Nimmagadda N, et al. Transurethral Anastomosis after Transurethral Radical Prostatectomy: A Phantom Study on Intraluminal Suturing With Concentric Tube Robots. IEEE Trans Med Robot Bionics 2020;2:578-81. [Crossref] [PubMed]
Cite this article as: Elbakry AA, Pan MM, Buckley JC. Frontiers in post-radiation urologic reconstruction; robotic surgery and near-infrared fluorescence imaging. AME Med J 2022;7:7.