Osteobiologics in degenerative spine disease: current clinical applications of recombinant human bone morphogenetic proteins—a narrative review
Introduction
Degenerative spine disease (DSD) represents a leading cause of disability as a primary driver of chronic low back and neck pain worldwide (1,2). The burden of these conditions is further expected to rise in the setting of an aging population. While conservative management may offer symptomatic relief, the progressive nature of degenerative spinal pathologies often necessitates operative intervention, wherein spinal fusions are a cornerstone (3). Amongst conventional procedures, bone grafts are commonly implemented to facilitate bone repair and regeneration, the current gold standard being iliac crest autograft, of which use is constrained by limited quantities and donor comorbidity (4,5). Such limitations in these bone grafts have prompted rising interest in more advanced osteobiologics of which recombinant human bone morphogenetic proteins (rhBMPs) have gained considerable traction in recent decades (6). In the context of spinal fusions, ideal grafting material should aim to mimic natural bone with regards to repair and regeneration. Alternatives to rhBMP, particularly synthetic osteobiologics such as calcium sulfates and phosphates, bioactive glass, and polymers, are not naturally occurring, giving rhBMP an osteoinductive edge in terms of emulating native bone. Additionally, rhBMPs address many practical and logistic drawbacks assumed with other grafting alternatives such as autografts, ceramics, bone marrow aspirate/stem cells, and demineralized bone matrix (DBM) given that they are reproducible, have long shelf-lives, and avoid risk of disease transmission.
The advent of rhBMP saw a preponderance of industry-sponsored studies to substantiate its osteoinductive efficacy in lieu of iliac bone crest autograft (ICBG) (7). With growing evidence to support its utility, rhBMP-2 (inFUSE, Medtronic, Inc., Minneapolis, MN, USA) received FDA approval for single-level anterior lumbar interbody fusion (ALIF) using a titanium tapered interbody cage (LT-CAGE, Medtronic, Inc.) first in 2002 (8), and subsequently in 2004 and 2009 with rhBMP-7 (OP-1, Stryker Biotech, Kalamazoo, MI, USA) and rhBMP-2 respectively, for revision posterolateral fusions (PLFs) within a select patient populations (9).
Upon initial inception in clinical practice, use of rhBMP-2 became increasingly prevalent, albeit most commonly through off-label use (10). Expanded applications across various spine procedures, particularly within anterior cervical discectomy and fusions (ACDFs), would reveal serious adverse events, inciting an FDA-issued black box warning in 2008 (11). In response, the safety profile of rhBMP-2 has since been called into question, with an array of reports documenting various inflammatory-mediated complications, heterotopic ossification, and neurological injury amongst others (11). Several studies have additionally described potential oncogenic effects with rhBMP-2 given lack of a regulatory mechanism within its pathway—the clinical data pertaining to its oncogenicity, however, remains speculative (11-13). Nonetheless, with further studies to repudiate safety concerns beyond ACDFs (14,15), utilization of rhBMP-2 for treatment of degenerative spine pathology remains prevalent in light of its osteoinductive capacity (16). This review provides an overview of rhBMP-2 applications in degenerative spine conditions, and summarizes current dispositions pertaining to its efficacy and safety profile within each context. We present this article in accordance with the Narrative Review reporting checklist (available at https://amj.amegroups.com/article/view/10.21037/amj-23-137/rc).
Methods
A literature search of the PubMed database was carried out in August 2023 to select papers published between 2000 and 2023 (Table 1). The inclusion criteria for source selection included studies that offered insights into the utilization, clinical outcomes, and potential adverse effects of rhBMP-2 use for the treatment of DSD and that were published in English or with translation. Articles that did not meet the above criteria were excluded. Included studies were based on a search query including the following MeSH terms “rhBMP”, “Spine Surgery”, “Spinal Fusion”, “Graft”. Primary source types targeted in the search included clinical trials, case reports, systematic reviews, and observational studies. After applying inclusion and exclusion criteria, 86 studies were selected.
Table 1
Items | Specification |
---|---|
Date of search | August 2023 |
Databases and other sources searched | PubMed database |
Search terms used | “rhBMP”, “Spine Surgery”, “Spinal Fusion”, “Graft" |
Timeframe | February 2000–August 2023 |
Inclusion and exclusion criteria | Inclusion criteria: studies reporting utilization, clinical outcomes, and potential adverse effects of rhBMP-2 in spine surgery for degenerative spine disease; articles published in English |
Exclusion criteria: studies that did not meet the above criteria; articles not published in English or without translation | |
Selection process | All authors conducted the search. Articles were included when authors reached consensus |
rhBMP-2, recombinant human bone morphogenetic protein-2.
Mechanistic background
BMPs belong to the transforming growth factor-beta (TGF-β) class of proteins integral for cell growth, differentiation, and development. Specifically, bone regeneration proceeds initially with interactions between two type I and type II serine/threonine kinase receptors on mesenchymal stem cells (17). Type II receptors subsequently phosphorylate type I glycine-serine (GS) domains, resulting in further downstream phosphorylation processes directly involved in bone growth, of which Smad1/5/8, mitogen-activated protein kinase (MAPK), and phosphatidylinositol 3-kinase (PI3K) are integral regulatory proteins (18).
The Smad pathway regulates osteogenic gene transcription, resulting in increased bone matrix protein production and mineralization, additionally driving chondrogenesis (19). The MAPK pathway has been implicated in bone remodeling pathways during regenerative processes, playing a critical role in both osteoblast proliferation and differentiation, as well as osteoclast regulation (20). On the other hand, PI3K facilitates osteoblast survival, growth, and differentiation, promoting cellular migration and adhesion which are crucial factors for effective bone repair and regeneration (21). As such, rhBMP offers a pragmatic clinical application in spinal fusion procedures primarily owed to their substantive role in bone regenerative signaling pathways, and consequently, their ability to augment bone formation (22). Along with non-specific differentiation of mesenchymal cells, the inherent absence of allosteric regulatory processes within the Smad pathway, however, leaves it susceptible to overactivation, which may potentially result in uncontrolled, downstream cellular proliferation. As such, the lack of a compensatory, negative feedback mechanism underlies clinical concerns regarding the oncogenicity of BMP as an osteobiologic (13). A brief illustration of the BMP intracellular signaling pathway is illustrated in Figure 1.
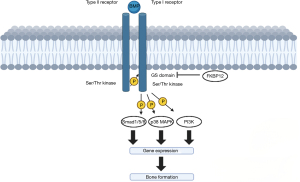
Food and Drug Administration (FDA)-approved applications
ALIF
The development of rhBMP-2 for use within spine surgery was notable as the first commercially available osteoinductive alternative autologous ICBG autograft (23). Boden et al. conducted a formative prospective randomized controlled trial (RCT) in 2000 in which rhBMP-2 was delivered using an absorbable collagen sponge (ACS) carrier and placed within cylindrical threaded fusion interbody cages (LT-CAGE, Medtronic, Inc.). Across their rhBMP-2 cohort, all patients demonstrated radiographic fusion, while only two of three ICBG patients had successful fusion (24). Drawing upon these preliminary reports, Burkus et al. performed a multicenter RCT which initially sought to establish non-inferiority, but instead observed significantly greater fusion rates in rhBMP-2 compared to ICBG (94.4% vs. 89.4%) at 2-year follow-up, and further improving Oswestry Disability Index (ODI) scores at 6-year follow-up (25)—these findings were followed by FDA-approval of the INFUSE bone graft (inFUSE, Medtronic, Inc.) in single-level ALIFs for lumbar degenerative disc disease (DDD). Burkus et al. further demonstrated improved clinical and radiographic outcomes using cortical allograft struts and the INTERFIXTM Threaded Fusion Device (Medtronic, Inc.) with rhBMP-2 compared to ICBG through a series of additional industry-sponsored studies (26,27). Similarly, Slosar et al. found the rhBMP-2 in combination with femoral ring allograft interbodies induced higher fusion rates than ICBG controls, with complete absence of revisions in the rhBMP-2 group and four (13%) within the autograft cohort (28).
In contrast to findings reported by industry-sponsored studies, Pradhan et al. reported greater nonunion rates with rhBMP-2 compared to ICBG (56% vs. 36%), wherein rhBMP-2 was also associated with significant femoral ring allograft resorption (29). Other studies moreover unveiled further evidence for increased endplate resorption, graft subsidence, and retrograde ejaculation with rhBMP-2 use (30-32).
Against the backdrop of contentious reports pertaining to rhBMP-2 use, the Yale University Open Data Access (YODA) Project synthesized data from Medtronic-sponsored trials with that of individual-participant data to conduct a comprehensive meta-analysis. Overall, findings from the YODA trial indicate equivalent fusion efficacy between rhBMP-2 and ICBG autograft, further reporting comparable incidence in neurological complications and retrograde ejaculation incidence following ALIFs (33). As such, single-level ALIFs using the inFUSETM Bone Graft, solely in combination with the LT-CAGETM tapered fusion cage, remains one of the few FDA-approved rhBMP-2 applications for treatment of lumbar degenerative pathology.
Revision PLFs
Amongst other FDA-approved applications for rhBMP, was its specific use within revision PLFs. In 2004, clinical trials revealed promising preliminary findings with rhBMP-7 or osteogenic protein-1 (OP-1, Stryker Biotech), subsequently prompting FDA-approved use in revision PLFs. Vaccaro et al. conducted a series of studies investigating the fusion efficacy of OP-1 putty relative to ICBG autograft indicated for degenerative lumbar spondylolisthesis, wherein the authors reported 86% of patients having attained clinical success with OP-1—defined as an improvement in preoperative ODI by ≥20%—compared to 73% using autograft. Similarly, radiographic fusion in OP-1 was observed in 74% of cases while only 60% in the autograft group (34,35). Although sample size limitations (n=36) precluded the study from statistical significance, findings nonetheless invited precursory conceptions that fusion rates and adverse effects between OP-1 putty and autograft were comparable (36). FDA-approved applications of OP-1 would expand to revision PLFs, albeit for select patient populations with compromised healing capabilities or in cases where autologous bone graft was inaccessible (37). Vaccaro et al. sought to expand upon previous preliminary studies in a prospective multicenter RCT including 295 patients, in which criteria for successful fusion was strictly modified to the presence of bridging bone on computed tomography (CT); subsequently, PLFs demonstrated a fusion success rate of 26% vs. 36% for autograft, invalidating the notion of non-inferiority between OP-1 and autograft in patients undergoing decompression and uninstrumented posterolateral lumbar fusion for single-level degenerative spondylolisthesis (38). These findings eventually led the FDA panel to reject premarket approval of OP-1 amongst other reasons pertaining to adverse effects noted with off-label applications (37).
In concurrence with OP-1, the INFUSE bone graft had also demonstrated substantial promise for use within PLF. Boden et al. conducted a prospective randomized multicenter trial comparing three groups of patients undergoing PLF for single-level DDD with various bone grafts including: (I) autograft with pedicle screw fixation, (II) rhBMP-2 with pedicle screw fixation, and (III) rhBMP-2 without. Across groups, the ICBG autograft group demonstrated a 40% fusion rate compared to 100% fusion rate for both rhBMP-2 cohorts, regardless of instrumentation, using a 20 mg dose per side (39). Similarly, Carreon et al. corroborated the efficacy of rhBMP-2 in single-level instrumented PLFs, reporting an 89% fusion rate with ICBG and 96% with rhBMP-2 at 2-year follow-up; reoperation rates were moreover significantly greater in the autograft group (16% vs. 8%), with 60% continuing to report persistent donor site pain (40).
Nonetheless, disparate reports were also documented. In 2010, Garrett et al. conducted retrospective review of 130 patients undergoing PLF using rhBMP-2, wherein 4.6% of patients subsequently developed sterile seromas requiring reoperation (41). One case report by Rower and Vickroy documented psoas ossification with L4–L5 PLF with subsequent reports of pain migration 3 months postoperatively (42).
Medtronic eventually sought FDA approval for use of higher rhBMP-2 dosages for an investigational device named AMPLIFY, to be used in PLF. In 2011, the FDA rejected the application due to concerns regarding the potential elevated risk of cancer development (43). In their meta-analysis, however, the 2013 YODA trial reported the risk of malignancy to be clinically insignificant, and further found that while rhBMP-2 carries a risk of ectopic bone formation, the assumed benefits outweigh the risks when used as an alternative to ICBG (33). More recently, Mariscal et al. [2020] conducted a meta-analysis overviewing rhBMP-2 vs. ICBG for lumbar spine PLF, which included 908 patients across six RCTs (446 rhBMP-2 vs. 462 ICBG). Fusion rates were significantly greater at both 6 months [86% vs. 60%; odds ratio (OR) =3.75; P<0.001] and 12 months [88% vs. 80%; OR =1.76; P=0.03] for rhBMP-2. The ICBG group was associated with significantly greater operative times and hospital length of stay (LOS), although patient-reported outcomes (PROs) including ODI and visual analogue scale (VAS) back pain scores, as well as adverse events were comparable (44). Notably, ICBG use was associated with a higher incidence of adverse events relative to rhBMP-2. Wu et al. [2021] substantiated these findings through a subsequent meta-analysis including 1,516 patients over 14 RCTs, reiterating the notion that rhBMP-2 conferred significantly higher fusion rates, lower operation times, and lower revision rates relative to conventional ICBGs (45). As such, the efficacy of rhBMP-2 in lumbar spine PSFs remains well-established despite the acknowledged, yet mitigable risk of potential adverse effects, lending to its utility as an FDA-approved treatment for degenerative spine conditions.
Off-label applications
ACDF
Amongst off-label applications for rhBMP-2, contentious perceptions were preeminently established following reports of serious complications in ACDFs. In 2006, Shields et al. performed a retrospective review involving high-dose inFUSE rhBMP-2 in ACDF, in which a greater incidence of postoperative complications, including hematoma, prolonged LOS, dysphagia, and respiratory distress was associated with greater dosages (46). Subsequently in 2007, Perri et al. documented a case of significant postoperative prevertebral soft-tissue swelling requiring intubation following two-level ACDF using rhBMP-2 (47); Vaidya et al. correspondingly noted significantly greater incidence in dysphagia at both 2- and 6-week postoperative marks in a separate consecutive case series comparing rhBMP-2 with polyetheretherketone (PEEK) cages and allograft spacers with DBM (48). Congruent reports to reveal the underlying inflammatory-mediated adverse effects would continue to arise (49,50), leading to an FDA-issued black box advisory in 2008 against the use of rhBMP-2/inFUSE in anterior cervical spine surgery (51). Contemporary use of rhBMP-2 in ACDF, however, continues to be prevalent, despite further reports to corroborate its adverse safety profile in line with FDA warnings (52,53).
Nonetheless, despite evolving perceptions since its inception, rhBMP-2 remains commonly implemented in clinical practice through off-label applications as a highly efficacious osteobiologic. In their pilot RCT comparing INFUSE Bone Graft and autogenous ICBG with CORNERSTONE-SR fibular allograft in 33 patients undergoing ACDF for degenerative cervical pathologies, Baskin et al. observed comparable fusion rates and safety profiles between cohorts, but significantly improved Neck Disability Index (NDI) and numerical rating scale (NRS) arm pain scores up to 24 months postoperatively. Within the ICBG group, statistically significant donor site pain continued to 6 weeks postoperatively, with residual cases persisting to 24 months (54). Regarding fusion efficacy, Frenkel et al. conducted a retrospective review of 45 patients undergoing multilevel ACDF for cervical DDD, and found a 100% successful fusion rate, determined using CT, with rhBMP-2 and 82.6% fusion rate for cases without (55). Tumialán et al. performed an observational cohort study involving 200 patients who underwent ACDF using rhBMP-2 with PEEK spacers and titanium plate fixation, which demonstrated 100% successful radiographic fusion across all cases (56). While greater risk for pseudarthrosis has been thoroughly associated with the number of fused levels, several studies have demonstrated the potential of rhBMP-2 to mitigate this risk (52,55). While continued use of rhBMP-2 in ACDF is predicated on its osteoinductive efficacy, the associated complication risks underlines the importance of judicious use—particularly in anticipation of compromised fusion quality.
Posterior cervical fusion (PCF)
Relative to ACDF, rhBMP-2 use in PCF reportedly assumes an improved safety profile. In 2009, Hiremath et al. performed a single-institution retrospective review of 83 patients who underwent PCF, either with or without rhBMP-2, and reported comparable rates of dysphagia and hematoma amongst other postoperative complications (57). Aligned to these findings, a more recent retrospective consecutive case series in 2022 of 765 patients by Weinberg et al. in 2022 reported a 96% successful fusion rate with rhBMP-2 vs. 91% for cases without one year postoperatively (P=0.02) (58). Another study, specifically within the context of subaxial DSD similarly revealed significant improvements in fusion rates with rhBMP-2 use (59).
Despite favorable features, some studies have suggested potential adverse effects of rhBMP-2 in PCF, reporting increased neck pain and risk of seroma formation (59,60). However, its overall safety has been accompanied by several large-scale studies attesting to similar complication profiles in PCFs performed with and without rhBMP-2 augmentation (60,61). Taken in constellation, current evidence serves to suggest that rhBMP-2 applications in PCF may enhance fusion rates without increasing risks of adverse clinical events as seen with ACDF.
Transforaminal lumbar interbody fusion (TLIF)
rhBMP-2 in the context of TLIF for DSD has demonstrated reliable fusion, however it has also been associated with adverse effects most commonly including radiculopathy, hematoma, and ectopic bone formation. A 2009 study done by Rihn et al., demonstrated radiographic fusion in 95.8% of cases in which rhBMP-2 was used for TLIF to treat DSD (62). Villavicencio et al. applied rhBMP-2 using an ACS to allografts used in TLIFs, demonstrating a 100% fusion rate at 12- and 24-month follow-up in 74 patients (63). Crandall et al. conducted an extensive study involving 509 patients undergoing TLIF with inFUSE with an average follow-up period of 59 months, revealing a 0.92% rate of pseudarthrosis and statistically significant enhancements in functional outcomes, including VAS and ODI scores at 1 and 2 years postoperatively (64). Other studies have demonstrated self-reported pain improvement and patient satisfaction rates of 83% and 94% respectively, in the context of TLIFs involving rhBMP-2 (62). Despite these favorable outcomes, several adverse effects of rhBMP-2 in TLIFs have been discussed in recent literature. In their study, Crandall et al. noted infrequent occurrences of seromas (0.4%) and ectopic bone growth (0.6%) within the rhBMP-2 cohort, which did not indicate a clear dose-related relationship with BMP; additionally, for patients with DSD treated using rhBMP-2, a 1.7% rate of deep infection was observed (64). In a retrospective review of 204 patients undergoing TLIF with rhBMP-2, Owens et al. observed complications in in 47 (21.6%) patients, with seroma/hematoma formation requiring revision in 4%, persistent radiculopathy in 2.9%, wound problems in 2.9%, and vertebral osteolysis in 0.5% (65). In addition, cases of rhBMP-2 in TLIF associated with ectopic bone formation leading to neurologic sequelae have been reported in the literature (66). Although rhBMP-2 is associated with favorable outcomes in TLIFs for DSD, these complication rates suggest cautious application of rhBMP-2 in this context.
Posterior lumbar interbody fusion (PLIF)
In the management of DSD with PLIF, rhBMP-2 has demonstrated promising outcomes, although it has raised significant concern with adverse effects that may have a role in tumor development. In a study evaluating rhBMP-2 in PLIF for patients with DSD, Meisel et al. observed full fusion demonstrated on 6-month post-op radiographs (67). A prospective study on PLIF conducted by Haid et al. found a 92.3% fusion rate for rhBMP-2 as compared to a 77.8% fusion rate for iliac crest autograft, although not statistically significant (68). With regards to pseudarthrosis, several studies have shown equivalent rates between PLIFs done with and without rhBMP-2 (69,70). In addition to successful fusion, rhBMP-2 has been associated with favorable functional outcomes in the context of PLIF. Haid et al. demonstrated significant improvements in ODI, back pain, leg pain, and physical components of SF-36 at all postoperative intervals for both rhBMP-2 and iliac autograft groups (68). Meisel et al. also demonstrated clear improvement in subjective pain measured by questionnaires at 24 months follow-up for patients who underwent PLIFs with rhBMP-2 (67). Despite successful clinical outcomes, studies have reported adverse effects of rhBMP-2 use in this context related to ectopic bone formation and subsequent neurological dysfunction (71). These complications allude to a potential oncogenic role of rhBMP-2, which has been a controversial topic of discussion.
Expanded applications: lateral lumbar interbody fusion (LLIF)/oblique lateral interbody fusion (OLIF)
In 2015, the FDA expanded applications of rhBMP-2 to include OLIFs, allowing the INFUSE Bone Graft to be used alongside two fusion devices: the Perimeter Interbody Fusion Device and the Clydesdale Spinal System. Now known as the INFUSETM Bone Graft/Medtronic Interbody Fusion Device, it was designed for spinal fusion procedures in patients diagnosed with DDD at a single level from L2–S1. With this new approval, the Clydesdale Spinal System could be implanted via an OLIF approach with rhBMP-2 at a single level from L2–L5. Another approved approach was implantation of the Perimeter Interbody Fusion Device through an OLIF approach with rhBMP-2 at a single level from L5–S1 (72,73). Despite the FDA’s approval for use of rhBMP-2 in very specific OLIF cases, its general application in both OLIFs and LLIFs lacks similar approval.
Despite lack of FDA-approval for general use in LLIFs and OLIFs for DSD, rhBMP-2 has demonstrated high fusion rates along with reduced blood loss, shortened hospitalization lengths, and reduced recovery times. In a study analyzing OLIFs done for DSD using rhBMP-2 incorporated into a PEEK cage, Kai et al. demonstrated a fusion rate of 100%, confirmed with radiographs (74). Singh et al. conducted a study exploring the efficacy of rhBMP-2 in LLIF procedures, revealing robust potential for achieving successful fusion outcomes in individuals with adult spinal deformity arising from degenerative spinal conditions (75). Another retrospective study compared three groups of 93 patients who underwent extreme lateral interbody fusions (XLIF), comparing group A: allogenic bone, group B: allogeneic bone + rhBMP-2, and group C: autologous bone marrow + allogeneic bone (76). It demonstrated a statistically significant increase in fusion rate for groups B and C as compared to group A, highlighting that the addition of rhBMP-2 to allogeneic bone yielded notable improvement in outcome. High fusion rates have been supported in a study by Nourian et al., which demonstrated fusion rates of 92% for single-level and 86% for two-level LLIFs using rhBMP-2 (77). In addition to fusion rates, studies incorporating rhBMP-2 have shown improved functional outcomes with long-term follow-up. Kai et al. had a mean follow up of 17.7 months and showed significantly improved back pain and leg pain VAS scores for their cohort of DSD patients undergoing OLIF using rhBMP-2 (74). Additionally, Gao et al. observed statistical improvement of VAS and ODI scores for all three groups, however, with higher improvement in group B (allogeneic bone + rhBMP-2) and C (autologous bone marrow + allogeneic bone) than group A (allogenic bone) (76). Given promising clinical outcomes, use of rhBMP-2 use is growing with regards to LLIF and OLIF management of DSD. However, few studies to date have explored its adverse effects when applied in this manner. Further analysis of long-term patient outcomes and safety profile is needed prior to widespread application of rhBMP-2 within this context, yet early studies demonstrate noteworthy potential.
Future directions
Delivery systems
Recent advancements in the clinical application of BMPs are now focusing largely on methods of delivery (78). Exploring synthetic osteobiologics such as ceramics and polymers as scaffolds for BMP delivery reveals synergistic effects, enhancing osteogenic properties and osteoblast differentiation (79,80). Particularly when applied with new 3D printing techniques, this combination of biologics has demonstrated success with fusion along with high biocompatibility and customizability (81). These advancements may enable us to construct individualized bone grafts with significant implications in regards to personalized medicine in spine surgery for degenerative disease. Targeted delivery systems also allow for precise application of BMP and better controlled dosing. In addition to osteobiologic scaffolds, novel delivery methods including viral vectors have demonstrated utility in BMP-2 gene transfer for bone regeneration in rat studies (82). Studies have shown the potential of viral vector gene therapy delivered through scaffolds to bypass biological and engineering barriers, offering promising applicability in vivo (83). Current research efforts are largely focused on expanding delivery options for BMPs with favorable preliminary data surrounding osteobiologics and viral vectors.
Small molecule osteogenic agents
Within the realm of bone grafting and regeneration, there are several newer molecules and substances that play important roles in the healing and integration of bone grafts. Oxysterols are one such molecule—they are osteoinductive agents with higher reproducibility and improved safety profiles (84). Studies have demonstrated an efficacy similar to that of rhBMPs at lower costs and with reduced adverse effects (85). However, given their early stage of development, extensive research is required before they can be widely clinically applied similarly to BMPs. In addition to oxysterols, growth factors have been a recent area of focus in the context of bone regeneration. Growth factors including vascular endothelial growth factor (VEGF), platelet-derived growth factor (PDGF), fibroblast growth factor (FGF), and insulin-like growth factor-1 (IGF-1) have well-established mechanisms in relation to tissue regeneration and angiogenesis. Recent literature has examined the utility of these growth factors when used alongside BMPs for bone growth and regeneration. These studies observed a synergistic effect between growth factors that enhance the osteoinductive properties of BMPs (86). Although knowledge of their safety profile is limited, current clinical evidence demonstrates a need for further exploration into these treatment options. These molecules demonstrated useful properties that, when used as an adjunct with BMPs, have great potential for bone growth and regeneration in the surgical management of DSD.
Study limitations
Although the objective of this review is to describe the contemporary evidence surrounding rhBMP-2 use in context of its historical timeline, it carries several limitations. As a narrative review, studies referenced herein were included on the basis that they yielded insight to the subject matter and contributed to the objectives of the present review. Studies included were therefore heterogeneous by design and level of evidence. It is important to highlight that the presented findings are intended to provide only a broad overview of the literature surrounding use of rhBMP-2 for degenerative spinal pathology rather than address a specified clinical question better suited through a systematic design. Given these limitations, this review presents an overview of rhBMP to provide context for its general clinical application rather than to guide clinical decision making. Caution should be exercised when using rhBMP-2 in a clinical setting with careful consideration to patient characteristics, comorbidities, and goals of treatment.
Conclusions
RhBMP-2 has become increasingly prevalent in treatment of DSD, despite limited FDA-approval. It is most commonly utilized in off-label procedures, highlighting the need for further research alongside caution with use. Despite promising osteogenic ability and high fusion rates, rhBMP-2 raises concerns with a range of adverse effects, most notably including seroma formation, retrograde ejaculation, ectopic bone formation, and a potential oncogenic role. As such, a comprehensive understanding of rhBMP-2 use for treatment of degenerative spine pathology is not only necessary to safely extend applications within spine surgery, but to further establish directions for forthcoming, innovative osteobiologic developments.
Acknowledgments
Funding: None.
Footnote
Reporting Checklist: The authors have completed the Narrative Review reporting checklist. Available at https://amj.amegroups.com/article/view/10.21037/amj-23-137/rc
Provenance and Peer Review: This article was commissioned by the Guest Editors (Mark Lambrechts and Brian Karamian) for the series “Degenerative Spine Disease” published in AME Medical Journal. The article has undergone external peer review.
Conflicts of Interest: All authors have completed the ICMJE uniform disclosure form (available at https://amj.amegroups.com/article/view/10.21037/amj-23-137/coif). The series “Degenerative Spine Disease” was commissioned by the editorial office without any funding or sponsorship. R.J.H. has received grant funding from SI bone, consulting fees from NuVasive, and support from the North American Spine Society to attend meetings. J.C.W. has received intellectual property royalties from Zimmer Biomet, NovApproach, SeaSpine, and DePuy Synthes, and is a committee member of AO Foundation. He holds stocks in the Bone Biologics, Electrocore, PearlDiver and Surgitech. R.K.A. has received grant funding from NIH, consulting fees from HIA Technologies, payment from Eccentrial Robotics for lectures and presentations, payment for expert testimony from Med-legal consulting (not testimony), support for attending meetings from USC, research support from Alphatec Spine, and holds stocks in the HIA Technologies. The authors have no other conflicts of interest to declare.
Ethical Statement: The authors are accountable for all aspects of the work in ensuring that questions related to the accuracy or integrity of any part of the work are appropriately investigated and resolved.
Open Access Statement: This is an Open Access article distributed in accordance with the Creative Commons Attribution-NonCommercial-NoDerivs 4.0 International License (CC BY-NC-ND 4.0), which permits the non-commercial replication and distribution of the article with the strict proviso that no changes or edits are made and the original work is properly cited (including links to both the formal publication through the relevant DOI and the license). See: https://creativecommons.org/licenses/by-nc-nd/4.0/.
References
- Hurwitz EL, Randhawa K, Yu H, et al. The Global Spine Care Initiative: a summary of the global burden of low back and neck pain studies. Eur Spine J 2018;27:796-801. [Crossref] [PubMed]
- Waheed MA, Hasan S, Tan LA, et al. Cervical spine pathology and treatment: a global overview. J Spine Surg 2020;6:340-50. [Crossref] [PubMed]
- Reisener MJ, Pumberger M, Shue J, et al. Trends in lumbar spinal fusion-a literature review. J Spine Surg 2020;6:752-61. [Crossref] [PubMed]
- Betz RR. Limitations of autograft and allograft: new synthetic solutions. Orthopedics 2002;25:s561-70. [Crossref] [PubMed]
- Schmidt AH. Autologous bone graft: Is it still the gold standard? Injury 2021;52:S18-22. [Crossref] [PubMed]
- Poon B, Kha T, Tran S, et al. Bone morphogenetic protein-2 and bone therapy: successes and pitfalls. J Pharm Pharmacol 2016;68:139-47. [Crossref] [PubMed]
- Hustedt JW, Blizzard DJ. The controversy surrounding bone morphogenetic proteins in the spine: a review of current research. Yale J Biol Med 2014;87:549-61. [PubMed]
- FDA. InFUSE™ Bone Graft/LT-CAGE™ Lumbar Tapered Fusion Device. Summary of Safety and Effectiveness Data Premarket Approval Application P000058. 2002.
- On Label and Off-Label Use of Bone Morphogenetic Proteins (BMPs). Accessed July 30, 2023. Available online: https://www.cms.gov/medicare-coverage-database/view/medcac-meeting.aspx?medcacid=55
- Ong KL, Villarraga ML, Lau E, et al. Off-label use of bone morphogenetic proteins in the United States using administrative data. Spine (Phila Pa 1976) 2010;35:1794-800. [Crossref] [PubMed]
- James AW, LaChaud G, Shen J, et al. A Review of the Clinical Side Effects of Bone Morphogenetic Protein-2. Tissue Eng Part B Rev 2016;22:284-97. [Crossref] [PubMed]
- Bobinac D, Marić I, Zoricić S, et al. Expression of bone morphogenetic proteins in human metastatic prostate and breast cancer. Croat Med J 2005;46:389-96. [PubMed]
- Skovrlj B, Koehler SM, Anderson PA, et al. Association Between BMP-2 and Carcinogenicity. Spine (Phila Pa 1976) 2015;40:1862-71. [Crossref] [PubMed]
- Zhang H, Wang F, Ding L, et al. A meta analysis of lumbar spinal fusion surgery using bone morphogenetic proteins and autologous iliac crest bone graft. PLoS One 2014;9:e97049. [Crossref] [PubMed]
- Cahill KS, Chi JH, Day A, et al. Prevalence, complications, and hospital charges associated with use of bone-morphogenetic proteins in spinal fusion procedures. JAMA 2009;302:58-66. [Crossref] [PubMed]
- Lykissas M, Gkiatas I. Use of recombinant human bone morphogenetic protein-2 in spine surgery. World J Orthop 2017;8:531-5. [Crossref] [PubMed]
- Cao X, Chen D. The BMP signaling and in vivo bone formation. Gene 2005;357:1-8. [Crossref] [PubMed]
- Katagiri T, Tsukamoto S. The unique activity of bone morphogenetic proteins in bone: a critical role of the Smad signaling pathway. Biol Chem 2013;394:703-14. [Crossref] [PubMed]
- Zhou N, Li Q, Lin X, et al. BMP2 induces chondrogenic differentiation, osteogenic differentiation and endochondral ossification in stem cells. Cell Tissue Res 2016;366:101-11. [Crossref] [PubMed]
- Ge C, Xiao G, Jiang D, et al. Critical role of the extracellular signal-regulated kinase-MAPK pathway in osteoblast differentiation and skeletal development. J Cell Biol 2007;176:709-18. [Crossref] [PubMed]
- Park YK, Heo SJ, Koak JY, et al. Characterization and Differentiation of Circulating Blood Mesenchymal Stem Cells and the Role of Phosphatidylinositol 3-Kinase in Modulating the Adhesion. Int J Stem Cells 2019;12:265-78. [Crossref] [PubMed]
- Beederman M, Lamplot JD, Nan G, et al. BMP signaling in mesenchymal stem cell differentiation and bone formation. J Biomed Sci Eng 2013;6:32-52. [Crossref] [PubMed]
- McKay WF, Peckham SM, Badura JM. A comprehensive clinical review of recombinant human bone morphogenetic protein-2 (INFUSE Bone Graft). Int Orthop 2007;31:729-34. [Crossref] [PubMed]
- Boden SD, Zdeblick TA, Sandhu HS, et al. The use of rhBMP-2 in interbody fusion cages. Definitive evidence of osteoinduction in humans: a preliminary report. Spine (Phila Pa 1976) 2000;25:376-81. [Crossref] [PubMed]
- Burkus JK, Gornet MF, Dickman CA, et al. Anterior lumbar interbody fusion using rhBMP-2 with tapered interbody cages. J Spinal Disord Tech 2002;15:337-49. [Crossref] [PubMed]
- Burkus JK, Heim SE, Gornet MF, et al. Is INFUSE bone graft superior to autograft bone? An integrated analysis of clinical trials using the LT-CAGE lumbar tapered fusion device. J Spinal Disord Tech 2003;16:113-22. [Crossref] [PubMed]
- Burkus JK, Sandhu HS, Gornet MF, et al. Use of rhBMP-2 in combination with structural cortical allografts: clinical and radiographic outcomes in anterior lumbar spinal surgery. J Bone Joint Surg Am 2005;87:1205-12. [Crossref] [PubMed]
- Slosar PJ, Josey R, Reynolds J. Accelerating lumbar fusions by combining rhBMP-2 with allograft bone: a prospective analysis of interbody fusion rates and clinical outcomes. Spine J 2007;7:301-7. [Crossref] [PubMed]
- Pradhan BB, Bae HW, Dawson EG, et al. Graft resorption with the use of bone morphogenetic protein: lessons from anterior lumbar interbody fusion using femoral ring allografts and recombinant human bone morphogenetic protein-2. Spine (Phila Pa 1976) 2006;31:E277-84. [Crossref] [PubMed]
- Vaidya R, Weir R, Sethi A, et al. Interbody fusion with allograft and rhBMP-2 leads to consistent fusion but early subsidence. J Bone Joint Surg Br 2007;89:342-5. [Crossref] [PubMed]
- Hansen SM, Sasso RC. Resorptive response of rhBMP2 simulating infection in an anterior lumbar interbody fusion with a femoral ring. J Spinal Disord Tech 2006;19:130-4. [Crossref] [PubMed]
- Carragee EJ, Mitsunaga KA, Hurwitz EL, et al. Retrograde ejaculation after anterior lumbar interbody fusion using rhBMP-2: a cohort controlled study. Spine J 2011;11:511-6. [Crossref] [PubMed]
- Simmonds MC, Brown JV, Heirs MK, et al. Safety and effectiveness of recombinant human bone morphogenetic protein-2 for spinal fusion: a meta-analysis of individual-participant data. Ann Intern Med 2013;158:877-89. [Crossref] [PubMed]
- Vaccaro AR, Patel T, Fischgrund J, et al. A pilot safety and efficacy study of OP-1 putty (rhBMP-7) as an adjunct to iliac crest autograft in posterolateral lumbar fusions. Eur Spine J 2003;12:495-500. [Crossref] [PubMed]
- Vaccaro AR, Patel T, Fischgrund J, et al. A pilot study evaluating the safety and efficacy of OP-1 Putty (rhBMP-7) as a replacement for iliac crest autograft in posterolateral lumbar arthrodesis for degenerative spondylolisthesis. Spine (Phila Pa 1976) 2004;29:1885-92. [Crossref] [PubMed]
- Vaccaro AR, Patel T, Fischgrund J, et al. A 2-year follow-up pilot study evaluating the safety and efficacy of op-1 putty (rhbmp-7) as an adjunct to iliac crest autograft in posterolateral lumbar fusions. Eur Spine J 2005;14:623-9. [Crossref] [PubMed]
- Munns J, Park DK, Singh K. Role of osteogenic protein-1/bone morphogenetic protein-7 in spinal fusion. Orthop Res Rev 2009;1:11-21. [Crossref]
- Vaccaro AR, Lawrence JP, Patel T, et al. The safety and efficacy of OP-1 (rhBMP-7) as a replacement for iliac crest autograft in posterolateral lumbar arthrodesis: a long-term (>4 years) pivotal study. Spine (Phila Pa 1976) 2008;33:2850-62. [Crossref] [PubMed]
- Boden SD, Kang J, Sandhu H, et al. Use of recombinant human bone morphogenetic protein-2 to achieve posterolateral lumbar spine fusion in humans: a prospective, randomized clinical pilot trial: 2002 Volvo Award in clinical studies. Spine (Phila Pa 1976) 2002;27:2662-73. [Crossref] [PubMed]
- Carreon LY, Glassman SD, Djurasovic M, et al. RhBMP-2 versus iliac crest bone graft for lumbar spine fusion in patients over 60 years of age: a cost-utility study. Spine (Phila Pa 1976) 2009;34:238-43. [Crossref] [PubMed]
- Garrett MP, Kakarla UK, Porter RW, et al. Formation of painful seroma and edema after the use of recombinant human bone morphogenetic protein-2 in posterolateral lumbar spine fusions. Neurosurgery 2010;66:1044-9; discussion 1049. [Crossref] [PubMed]
- Brower RS, Vickroy NM. A case of psoas ossification from the use of BMP-2 for posterolateral fusion at L4-L5. Spine (Phila Pa 1976) 2008;33:E653-5. [Crossref] [PubMed]
- Carragee EJ, Hurwitz EL, Weiner BK. A critical review of recombinant human bone morphogenetic protein-2 trials in spinal surgery: emerging safety concerns and lessons learned. Spine J 2011;11:471-91. [Crossref] [PubMed]
- Mariscal G, Nuñez JH, Barrios C, et al. A meta-analysis of bone morphogenetic protein-2 versus iliac crest bone graft for the posterolateral fusion of the lumbar spine. J Bone Miner Metab 2020;38:54-62. [Crossref] [PubMed]
- Wu Z, Zhou B, Chen L, et al. Bone morphogenetic protein-2 against iliac crest bone graft for the posterolateral fusion of the lumbar spine: A meta-analysis. Int J Clin Pract 2021;75:e13911. [Crossref] [PubMed]
- Shields LB, Raque GH, Glassman SD, et al. Adverse effects associated with high-dose recombinant human bone morphogenetic protein-2 use in anterior cervical spine fusion. Spine (Phila Pa 1976) 2006;31:542-7. [Crossref] [PubMed]
- Perri B, Cooper M, Lauryssen C, et al. Adverse swelling associated with use of rh-BMP-2 in anterior cervical discectomy and fusion: a case study. Spine J 2007;7:235-9. [Crossref] [PubMed]
- Vaidya R, Carp J, Sethi A, et al. Complications of anterior cervical discectomy and fusion using recombinant human bone morphogenetic protein-2. Eur Spine J 2007;16:1257-65. [Crossref] [PubMed]
- Shahlaie K, Kim KD. Occipitocervical fusion using recombinant human bone morphogenetic protein-2: adverse effects due to tissue swelling and seroma. Spine (Phila Pa 1976) 2008;33:2361-6. [Crossref] [PubMed]
- Smucker JD, Rhee JM, Singh K, et al. Increased swelling complications associated with off-label usage of rhBMP-2 in the anterior cervical spine. Spine (Phila Pa 1976) 2006;31:2813-9. [Crossref] [PubMed]
- Epstein NE. Complications due to the use of BMP/INFUSE in spine surgery: The evidence continues to mount. Surg Neurol Int 2013;4:S343-52. [Crossref] [PubMed]
- Buttermann GR. Prospective nonrandomized comparison of an allograft with bone morphogenic protein versus an iliac-crest autograft in anterior cervical discectomy and fusion. Spine J 2008;8:426-35. [Crossref] [PubMed]
- Kukreja S, Ahmed OI, Haydel J, et al. Complications of Anterior Cervical Fusion using a Low-dose Recombinant Human Bone Morphogenetic Protein-2. Korean J Spine 2015;12:68-74. [Crossref] [PubMed]
- Baskin DS, Ryan P, Sonntag V, et al. A prospective, randomized, controlled cervical fusion study using recombinant human bone morphogenetic protein-2 with the CORNERSTONE-SR allograft ring and the ATLANTIS anterior cervical plate. Spine (Phila Pa 1976) 2003;28:1219-24; discussion 1225. [Crossref] [PubMed]
- Frenkel MB, Cahill KS, Javahary RJ, et al. Fusion rates in multilevel, instrumented anterior cervical fusion for degenerative disease with and without the use of bone morphogenetic protein. J Neurosurg Spine 2013;18:269-73. [Crossref] [PubMed]
- Tumialán LM, Pan J, Rodts GE, et al. The safety and efficacy of anterior cervical discectomy and fusion with polyetheretherketone spacer and recombinant human bone morphogenetic protein-2: a review of 200 patients. J Neurosurg Spine 2008;8:529-35. [Crossref] [PubMed]
- Hiremath GK, Steinmetz MP, Krishnaney AA. Is it safe to use recombinant human bone morphogenetic protein in posterior cervical fusion? Spine (Phila Pa 1976) 2009;34:885-9. [Crossref] [PubMed]
- Weinberg DS, Eoh JH, Manz WJ, et al. Off-label usage of RhBMP-2 in posterior cervical fusion is not associated with early increased complication rate and has similar clinical outcomes. Spine J 2022;22:1079-88. [Crossref] [PubMed]
- Xu R, Bydon M, Sciubba DM, et al. Safety and efficacy of rhBMP2 in posterior cervical spinal fusion for subaxial degenerative spine disease: Analysis of outcomes in 204 patients. Surg Neurol Int 2011;2:109. [Crossref] [PubMed]
- Wanderman NR, Drayer NJ, Tomov M, et al. Postoperative Seroma Formation After Posterior Cervical Fusion with Use of RhBMP-2: A Report of Two Cases. JBJS Case Connect 2018;8:e74. [Crossref] [PubMed]
- Hodges SD, Eck JC, Newton D. Retrospective study of posterior cervical fusions with rhBMP-2. Orthopedics 2012;35:e895-8. [Crossref] [PubMed]
- Rihn JA, Makda J, Hong J, et al. The use of RhBMP-2 in single-level transforaminal lumbar interbody fusion: a clinical and radiographic analysis. Eur Spine J 2009;18:1629-36. [Crossref] [PubMed]
- Villavicencio AT, Burneikiene S. RhBMP-2-induced radiculitis in patients undergoing transforaminal lumbar interbody fusion: relationship to dose. Spine J 2016;16:1208-13. [Crossref] [PubMed]
- Crandall DG, Revella J, Patterson J, et al. Transforaminal lumbar interbody fusion with rhBMP-2 in spinal deformity, spondylolisthesis, and degenerative disease—part 2: BMP dosage-related complications and long-term outcomes in 509 patients. Spine (Phila Pa 1976) 2013;38:1137-45. [Crossref] [PubMed]
- Owens K, Glassman SD, Howard JM, et al. Perioperative complications with rhBMP-2 in transforaminal lumbar interbody fusion. Eur Spine J 2011;20:612-7. [Crossref] [PubMed]
- Chen NF, Smith ZA, Stiner E, et al. Symptomatic ectopic bone formation after off-label use of recombinant human bone morphogenetic protein-2 in transforaminal lumbar interbody fusion. J Neurosurg Spine 2010;12:40-6. [Crossref] [PubMed]
- Meisel HJ, Schnöring M, Hohaus C, et al. Posterior lumbar interbody fusion using rhBMP-2. Eur Spine J 2008;17:1735-44. [Crossref] [PubMed]
- Haid RW Jr, Branch CL Jr, Alexander JT, et al. Posterior lumbar interbody fusion using recombinant human bone morphogenetic protein type 2 with cylindrical interbody cages. Spine J 2004;4:527-38; discussion 538-9. [Crossref] [PubMed]
- Singh K, Ahmadinia K, Park DK, et al. Complications of spinal fusion with utilization of bone morphogenetic protein: a systematic review of the literature. Spine (Phila Pa 1976) 2014;39:91-101. [Crossref] [PubMed]
- Lubelski D, Abdullah KG, Steinmetz MP, et al. Adverse Events With the Use of rhBMP-2 in Thoracolumbar and Lumbar Spine Fusions: A 9-Year Institutional Analysis. J Spinal Disord Tech 2015;28:E277-83. [Crossref] [PubMed]
- Wong DA, Kumar A, Jatana S, et al. Neurologic impairment from ectopic bone in the lumbar canal: a potential complication of off-label PLIF/TLIF use of bone morphogenetic protein-2 (BMP-2). Spine J 2008;8:1011-8. [Crossref] [PubMed]
- Epstein NE. Pros, cons, and costs of INFUSE in spinal surgery. Surg Neurol Int 2011;2:10. [Crossref] [PubMed]
- BlueCross BlueShield of North Carolina. Bone Morphogenetic Protein. BCBSNC. Origination:
10/2014. Last Review: 2/2023. Available online: https://www.bluecrossnc.com/sites/default/files/document/attachment/services/public/pdfs/medicalpolicy/bone_morphogenetic_protein.pdf - Kai W, Cheng C, Yao Q, et al. Oblique Lumbar Interbody Fusion Using a Stand-Alone Construct for the Treatment of Adjacent-Segment Lumbar Degenerative Disease. Front Surg 2022;9:850099. [Crossref] [PubMed]
- Singh V, Oppermann M, Evaniew N, et al. Lateral Lumbar Interbody Fusion With rhBMP-2 can Achieve High Fusion Rates in Adult Spine Deformity Surgeries. Global Spine J 2024;14:244-56. [Crossref] [PubMed]
- Gao Y, Li J, Cui H, et al. Comparison of intervertebral fusion rates of different bone graft materials in extreme lateral interbody fusion. Medicine (Baltimore) 2019;98:e17685. [Crossref] [PubMed]
- Nourian AA, Harrington J, Pulido PA, et al. Fusion Rates of Lateral Lumbar Interbody Fusion Using Recombinant Human Bone Morphogenetic Protein-2. Global Spine J 2019;9:398-402. [Crossref] [PubMed]
- Liu Q, Peng X, Liu X, et al. Advances in the application of bone morphogenetic proteins and their derived peptides in bone defect repair. Compos B Eng 2023;262:110805. [Crossref]
- Gan Q, Pan H, Zhang W, et al. Fabrication and evaluation of a BMP-2/dexamethasone co-loaded gelatin sponge scaffold for rapid bone regeneration. Regen Biomater 2022;9:rbac008. [Crossref] [PubMed]
- Schlickewei C, Klatte TO, Wildermuth Y, et al. A bioactive nano-calcium phosphate paste for in-situ transfection of BMP-7 and VEGF-A in a rabbit critical-size bone defect: results of an in vivo study. J Mater Sci Mater Med 2019;30:15. [Crossref] [PubMed]
- Li Z, Wang Q, Liu G. A Review of 3D Printed Bone Implants. Micromachines (Basel) 2022;13:528. [Crossref] [PubMed]
- Kawai MY, Ozasa R, Ishimoto T, et al. Periodontal Tissue as a Biomaterial for Hard-Tissue Regeneration following bmp-2 Gene Transfer. Materials (Basel) 2022;15:993. [Crossref] [PubMed]
- Madrigal JL, Stilhano R, Silva EA. Biomaterial-Guided Gene Delivery for Musculoskeletal Tissue Repair. Tissue Eng Part B Rev 2017;23:347-61. [Crossref] [PubMed]
- Johnson JS, Meliton V, Kim WK, et al. Novel oxysterols have pro-osteogenic and anti-adipogenic effects in vitro and induce spinal fusion in vivo. J Cell Biochem 2011;112:1673-84. [Crossref] [PubMed]
- Hokugo A, Sorice S, Parhami F, et al. A novel oxysterol promotes bone regeneration in rabbit cranial bone defects. J Tissue Eng Regen Med 2016;10:591-9. [Crossref] [PubMed]
- Bayer EA, Fedorchak MV, Little SR. The Influence of Platelet-Derived Growth Factor and Bone Morphogenetic Protein Presentation on Tubule Organization by Human Umbilical Vascular Endothelial Cells and Human Mesenchymal Stem Cells in Coculture. Tissue Eng Part A 2016;22:1296-304. [Crossref] [PubMed]
Cite this article as: Shah I, Chopra M, Ton A, Hah RJ, Wang JC, Alluri RK. Osteobiologics in degenerative spine disease: current clinical applications of recombinant human bone morphogenetic proteins—a narrative review. AME Med J 2024;9:5.