Modifications in gamma-aminobutyric acid type A receptor subunit gene expression after macrophage differentiation and propofol administration in THP-1 cells
Highlight box
Key findings
• Gene expressions of α1 and α4 (which have anti-inflammatory effect) gamma-aminobutyric acid type A (GABAA) receptor subunits increased following propofol administration after differentiation into inflammatory macrophage-like THP-1 cells. GABAA receptors can be involved in the immunosuppressive effects of propofol.
What is known and what is new?
• Propofol has an immunosuppressive effect. However, the precise mechanism of immunosuppressive effects is unknown. Propofol has hypnotic activity through the activation of GABAA receptors. The GABAergic signaling system is present in immune cells.
• The gene expression of GABAA receptor subunits was modified after macrophage differentiation in THP-1 cells. The gene expression of α1 and α4 subunits was increased by propofol administration after macrophage differentiation.
What is the implication, and what should change now?
• Propofol can exert an anti-inflammatory effect through the GABAA receptor. The findings of this study can aid clinicians in the choice of anesthetic agents for proinflammatory conditions.
Introduction
Background
Gamma-aminobutyric acid type A (GABAA) receptors are ligand-gated anion channels activated when gamma-aminobutyric acid (GABA) binds to them. GABA is a major inhibitory neurotransmitter. The function of GABA has been well studied in the central nervous system; however, few studies are available on GABAA receptor subunit expression in relation to physiological functions in other tissues. In particular, the expression of GABAA receptors and their functions in immune cells have not been fully investigated. GABAA receptor expression has been confirmed in monocytes (1), human acute monocytic leukemia cell lines (THP-1 cells), macrophages (2,3), and T-cells (4,5). Monocytes and macrophages produce GABA, inhibiting inflammatory cytokine production (6-8). Hence, it has been hypothesized that the GABAergic signaling system is also present in immune cells, regulating cellular functions such as cell proliferation, cytokine production, phagocytosis, and chemotaxis (2,6,8,9).
The GABAA receptor is a pentamer consisting of three different subunits. There are 19 different types of GABAA receptor subunits (α1–6, β1–3, γ1–3, δ, ε, π, θ, and ρ1–3). GABAA receptors are mainly composed of 2α, 2β, and 1γ or 1δ subunits (10). Since the difference in composition determines the channel’s specific function and pharmacological properties, identifying subunit expression in cells is crucial. The hypnotic effect of anesthetics is produced by the GABAA receptors (11). Propofol, a predominant hypnotic agent in the anesthetic field, is known to act on immune cells, resulting in immunosuppressive effects (12,13).
Rationale and knowledge gap
Macrophages are immune cells that are present in various tissues throughout the body. Monocytes differentiate into macrophages when they migrate to tissues throughout the body. During inflammatory processes, macrophages differentiate into M1 macrophages, which act as inflammatory cells, and M2 macrophages, responsible for tissue repair. These macrophages play an important role in the immune response (14,15). THP-1, a human acute monocytic leukemia cell line, has been widely used to investigate the function of human macrophages. THP-1 cells can differentiate into macrophage-like and M1/M2 macrophage-like cells (16,17). In a previous study using THP-1-derived macrophages, we found that propofol suppresses interleukin (IL)-6 and IL-1β production without affecting M1/M2 differentiation and that GABAA receptors could be involved in the suppression of cytokine production (18).
The expression of GABAA receptor subunits on T cells and monocytes can be altered by influenza infection. The administration of diazepam affects immune function and increases susceptibility to infection (19). This finding indicates that changes in GABAA receptor subunit expression are involved in immune function and exacerbate the immunosuppressive effect of diazepam.
Objective
Since the GABAA subunits expressed in immune cells during inflammation have not been fully investigated, we aimed to analyze the expression of GABAA receptor subunit genes in THP-1 cells after macrophage differentiation. Furthermore, we aimed to investigate the changes induced by propofol administration to clarify the precise mechanism of the immunosuppressive effect of propofol. We present this article in accordance with the MDAR reporting checklist (available at https://amj.amegroups.com/article/view/10.21037/amj-23-58/rc).
Methods
Materials
Roswell Park Memorial Institute (RPMI) 1640 medium, dimethyl sulfoxide (DMSO), lipopolysaccharide (LPS) from Escherichia coli strain O111:B4, and phorbol-12-myristate-13-acetate (PMA) were obtained from Sigma-Aldrich (St. Louis, MO, USA). Interferon (IFN)-γ was obtained from R&D Systems (Minneapolis, MN, USA). Propofol was obtained from Wako Pure Chemical Industries (Osaka, Japan).
Cell culture and differentiation
We used the following cell line: (RRID:CVCL 0006). THP-1 cells (ATCC; Manassas, VA, USA) resemble primary monocytes and macrophages in morphology and differentiation properties. When exposed to PMA, THP-1 cells adhere to culture plates and start differentiating into a macrophage-like phenotype; these cells are generally used to study human macrophage functions (16). THP-1 cells were differentiated into macrophage-like cells (M0 THP-1) through incubation for 3 days with 200 nM PMA in RPMI 1640 supplemented with 5% fetal bovine serum (FBS), penicillin (100 IU/mL), and streptomycin (100 µg/mL) (16). M0 THP-1 cells were polarized into inflammatory macrophage-like cells (M1 THP-1) via incubation with 100 ng/mL of LPS and 20 ng/mL of IFN-γ for 18 h (17). We confirmed the appropriate differentiation into M0 and M1 macrophages by analyzing the differentiation marker gene expressions under our experimental conditions. Cluster of differentiation (CD)68 (macrophage marker) gene expression was upregulated approximately 1.5-fold when THP-1 cells were differentiated into M0 THP-1 cells. CD86 (M1 marker) gene expression was upregulated approximately 2.0-fold when M0 THP-1 cells were differentiated into M1 THP-1 cells (Figure 1).
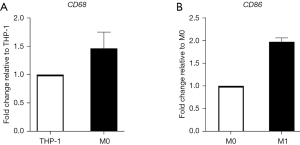
M0 THP-1 cells were differentiated into M1 THP-1 cells in the presence of propofol (25–100 µM) or in that of the solvent alone (0.05% DMSO) to evaluate the effects of propofol on GABAA subunit gene expression after M1 differentiation. Under these experimental conditions, propofol had little effect on the viability of polarized THP-1 cells. Cell viability measured by trypan blue staining was 97.9%±3.6%, 96.6%±2.8%, and 96.6%±1.2% for propofol concentrations of 25, 50, and 100 µM, respectively when control was 100% (Figure 2).
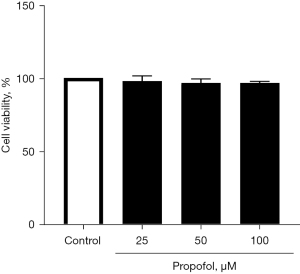
Quantitative reverse transcription polymerase chain reaction (qRT-PCR) assays
Total cellular RNA was extracted using the RNeasy Mini Kit (Qiagen, Valencia, CA, USA) according to the manufacturer’s instructions. Subsequently, complementary DNA (cDNA) was synthesized from the total RNA preparations using a high-capacity cDNA reverse transcription kit (Applied Biosystems, Foster City, CA, USA) by following the guidelines. Furthermore, qRT-PCR was performed using PowerUp SYBR Green Master Mix (Applied Biosystems) and specific primers (Takara, Kusatsu, Japan; Table 1) on a 7500 Fast Real-Time PCR System (Applied Biosystems). Target messenger RNA (mRNA) levels were normalized against housekeeping β-actin mRNA levels, and the expression level relative to that of the control was calculated using the ∆∆Ct method. The relative mRNA expression was expressed as fold expression over the control gene expression. The expression level with the control treatment was assumed to be 1.
Table 1
Gene | Forward primer (5'→3') | Reverse primer (5'→3') |
---|---|---|
β-actin | TGGCACCCAGCACAATGAA | CTAAGTCATAGTCCGCCTAGAAGCA |
α1 | CAGCAAGTATGAACCACATGGAAC | ATGTGTGGAATGACTTGAACAGAGA |
α2 | TCCCAAGTTTCATTCTGGCTTAACA | ACCAGTCCATGGCAGTTGCATA |
α3 | GTCACAAGTGTCGTTCTGGCTCA | AGTCCATGGCCGTCGCATA |
α4 | GTGCAGCATGTTGGCTTGTC | TCGCATAGATACACCTTTGCATGA |
α5 | ATCTTGGATGGGCTCTTGGATG | CCGAAGCTGGTGACGTAGATG |
α6 | AGGAGTCCGTCCCAGCAAGA | GTTGACAGCTGCGAACTCGATAAG |
β1 | TCTGCAGCCAGAGTCGCACTA | ATACTCCAGCAGAGCCAGGAACA |
β2 | CTTTGAGTTCCCAAACCAAATGTC | TGGAACTGTCAACTTGCTTCAAATG |
β3 | GCAGAACTGCACTCTGGAAATTGA | TCCACTCCGGTAACAGCCTTG |
γ1 | GCAGCCTTGATGGAATATGGAAC | TGGATCCAGGATGGAGACCAG |
γ2 | TCTGGCAAATCTCTGTGCTG | TCACTTGACAACACCTATGTGAGAA |
γ3 | TGGATCACCACACCCAATCAG | ATCAGCGGGCAGGAGTGTTC |
δ | GTGCATGCTGGACCTGGAGA | CGGTAGCTGGTGATGGTGAAC |
CD68 | TTGCAGCAACTCGAGCATCA | CAGCAAGATGGACCGGTCAC |
CD86 | CTGTAACTCCAGCTCTGCTCCGTA | GCCCATAAGTGTGCTCTGAAGTGA |
RT-PCR, reverse transcription polymerase chain reaction.
Statistical analysis
Values are expressed as the mean ± standard deviation (SD), and the results were obtained from six separate experiments. While differences between two groups were analyzed using an unpaired two-tailed t-test, differences among multiple groups were analyzed using a one-way analysis of variance (ANOVA), followed by Bonferroni’s post-hoc test. All statistical analyses were performed using GraphPad Prism software program V. 6.00 (GraphPad Software; La Jolla, CA, USA), with P<0.05 defined as statistically significant.
Ethical consideration
The study was conducted in accordance with the Declaration of Helsinki (as revised in 2013). This study used THP-1 cells of the cell line which were commercially available in cell culture experiments, not with human primary cells. The ethics registration and informed consent for this study were waived.
Results
Increase in the gene expression of α1, α4, β1, β2, and γ2 GABAA receptor subunits after differentiation into M0 THP-1 cells
GABAA receptor subunit gene expression was investigated after macrophage differentiation. The gene expression of α1, α4, β1, β2, and γ2 GABAA receptor subunits in THP-1 cells significantly increased after the differentiation into M0 THP-1 cells. The expression of α3, α5, α6, β3, and γ3 was not detected in THP-1 cells. The expression of α2 and δ did not change after macrophage differentiation (Figure 3).
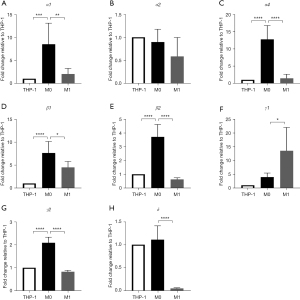
Decrease in the expression of α1, α4, β1, β2, γ2, and δ subunits and increase in the expression of γ1 subunit after differentiation into M1 THP-1 cells
We examined GABAA receptor subunit gene expression in THP-1 cells after M1 differentiation. The gene expression of α1, α4, β1, β2, γ2, and δ decreased significantly after the differentiation into M1 THP-1 cells, while that of γ1 showed a significant increase (Figure 3).
Increase in the expression of α1, α4, and β2 GABAA receptor subunits following propofol administration after differentiation into M1 THP-1 cells
We evaluated the effect of propofol on the expression of GABAA receptor subunits after M1 differentiation in THP-1 cells. Propofol significantly increased the gene expression of α1, α4, and β2, but did not affect the expression of β1, γ1, γ2, or δ (Figure 4).
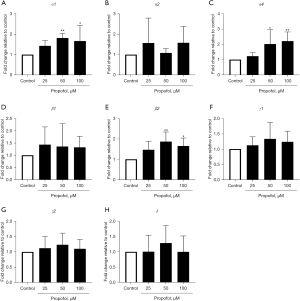
Discussion
Key findings
In this study, we detected the gene expression of α1, α2, α4, β1, β2, γ1, γ2, and δ GABAA receptor subunits in THP-1 cells. The gene expression of α1, α4, β1, β2, γ1, and γ2 increased after macrophage differentiation. The gene expression of α1, α4, β1, β2, γ2, and δ decreased after M1 differentiation; additionally, propofol administration increased the expression of α1, α4, and β2 after M1 differentiation.
Strengths and limitations
We suggested that THP-1 cells actually express several GABAA receptor subunits gene expression, which is altered after macrophage differentiation and propofol administration. These changes can be the mechanism of propofol’s immunosuppressive effects.
This study has several limitations. First, an artificial THP-1 cell line was used; therefore, macrophages from human peripheral blood should be examined in a clinical setting in a future study. This is because the results from peripheral blood more likely resemble those acquired in actual situations and may easily be applied in clinical therapy. Second, only gene expression was analyzed in this study; therefore, changes should be examined at the protein level to clarify the precise mechanism of the immunosuppressive effect via the GABAA receptor. Third, we analyzed only 13 major subunits; however, all 19 GABAA receptor subunits should be investigated in order to acquire more accurate results. Further studies are required to address these limitations.
Comparison with similar research
GABA is a major inhibitory neurotransmitter in the mammalian central nervous system. GABAA receptors, the primary target of GABA, are pentameric complexes consisting of three different subunits. Various combinations of GABAA receptor subunits determine receptor function. Mammals express 20–30 different GABAA receptor isoforms. The most common combination of GABAA receptor subunits consists of two α subunits, two β subunits, and one γ or δ subunit (20). In this study, we investigated 13 GABAA receptor subunits: α1–6, β1–3, γ1–3, and δ. Most of the hypnotic effects of anesthetic agents are produced by activating GABAA receptors. Classical benzodiazepines, such as diazepam, bind to two distinct binding sites on this receptor. They may bind to the α-γ subunit interface in the receptor’s extracellular domain. Benzodiazepines may also bind to the β-α and γ-β interfaces, which are present in the transmembrane domain. Propofol binds to the β-α interface in the transmembrane domain of the receptor (21).
GABAA receptors are present in immune cells, and GABAA signaling modifies immune function. GABAA receptors have been found on CD4+ and CD8+ T cells, macrophages, monocytes, and THP-1 cells (1-5). The administration of diazepam in a mouse pneumonia model leads to immunosuppression, resulting in higher mortality. In contrast, administering of the GABAA receptor antagonist, bicuculline counteracts the immunosuppressive effect of diazepam and decreases mortality (22). Another study reported that benzodiazepines are involved in immunosuppressive effects, resulting in increased mortality and the incidence of pneumonia (23). The expression of GABAA receptor subunits on T cells and monocytes is modified by influenza infection, and diazepam administration affects immune function and increases susceptibility to infection (19). These results indicate that the expression of GABAA receptor subunits can be modified by external stimuli, such as inflammation, differentiation, and drug administration, including the administration of intravenous anesthetics.
Explanations of findings
Our previous study showed that via the effects on GABAA receptors in THP-1 cells, propofol suppresses the production of inflammatory cytokines, IL-6 and IL-1β, after the differentiation into inflammatory M1 macrophage-like cells without affecting M1 differentiation (18). The present study shows that the gene expression of α1, α4, β2, and other subunits of GABAA receptors decreased after M1 differentiation. Conversely, the addition of propofol increased the gene expression of α1, α4, and β2 subunits after M1 differentiation. Combined, our previous and present data suggest that the suppression of inflammatory cytokines production after M1 differentiation in THP-1 cells by propofol administration may be associated with increasing the gene expression of α1, α4, and β2 subunits.
GABAA receptors are reportedly involved in inflammatory diseases, such as asthma, intestinal inflammation, and pulmonary fibrosis. The GABAA receptor, α1, is observed in human alveolar macrophages and monocytes and is responsible for diazepam-induced immunosuppression (19,22). When the GABAA receptor, α4, is knocked out in mice suffering from asthma, lung inflammation and airway reactivity deteriorate further (24). In mice with stress-induced intestinal inflammation, α1, α4, and α5 GABAA receptor agonists exert anti-inflammatory effects, while α3 receptor agonists exacerbate inflammation (25). Diazepam administration activates GABAAα4 receptors, suppressing LPS-induced lung injury and the development of pulmonary fibrosis (26). These results suggest that α1 and α4 GABAA receptors are involved in suppressing inflammatory responses. In addition to such previous data, our data suggest that propofol suppresses the production of inflammatory cytokines in THP-1 cells after the M1 differentiation and increases gene expressions of α1, α4, and β2 subunits of GABAA receptors after M1 differentiation. Considering these findings together, it is quite possible that propofol exerts its immunosuppressive effect by increasing the expressions of the α1 and α4 subunits of GABAA receptors.
Implications and actions needed
In this study, we suggested the gene expression of GABAA receptor subunits was changed after macrophage differentiation and propofol administration in THP-1 cells. The gene expression of α1 and α4 subunits which are involved in anti-inflammatory effects was increased by propofol administration. Therefore, the immunosuppressive effect of propofol may be related to changes in gene expressions. Propofol can be beneficial in highly inflammatory situations.
Conclusions
In this study, the gene expression of GABAA receptor subunits was modified after macrophage differentiation in THP-1 cells. In particular, propofol administration increased the gene expression of α1 and α4 subunits after M1 differentiation. These results suggest that the immunosuppressive effect of propofol may be related to changes in the gene expression of GABAA receptor subunits. These results can help clinicians choose appropriate anesthetic agents for proinflammatory treatments, such as invasive surgery.
Acknowledgments
We would like to thank the Laboratory of Molecular and Biochemical Research, Research Support Center, Juntendo University Graduate School of Medicine, for technical assistance. Additionally, we would like to thank Editage (https://www.editage.com/) for English language editing.
Funding: This work was supported by
Footnote
Reporting Checklist: The authors have completed the MDAR reporting checklist. Available at https://amj.amegroups.com/article/view/10.21037/amj-23-58/rc
Data Sharing Statement: Available at https://amj.amegroups.com/article/view/10.21037/amj-23-58/dss
Peer Review File: Available at https://amj.amegroups.com/article/view/10.21037/amj-23-58/prf
Conflicts of Interest: All authors have completed the ICMJE uniform disclosure form (available at https://amj.amegroups.com/article/view/10.21037/amj-23-58/coif). I.K. serves as an unpaid editorial board member of AME Medical Journal from February 2023 to January 2025. The other authors have no conflicts of interest to declare.
Ethical Statement: The authors are accountable for all aspects of the work in ensuring that questions related to the accuracy or integrity of any part of the work are appropriately investigated and resolved. The study was conducted in accordance with the Declaration of Helsinki (as revised in 2013). This study used THP-1 cells of the cell line which were commercially available in cell culture experiments, not with human primary cells. The ethics registration and informed consent for this study were waived.
Open Access Statement: This is an Open Access article distributed in accordance with the Creative Commons Attribution-NonCommercial-NoDerivs 4.0 International License (CC BY-NC-ND 4.0), which permits the non-commercial replication and distribution of the article with the strict proviso that no changes or edits are made and the original work is properly cited (including links to both the formal publication through the relevant DOI and the license). See: https://creativecommons.org/licenses/by-nc-nd/4.0/.
References
- Alam S, Laughton DL, Walding A, et al. Human peripheral blood mononuclear cells express GABAA receptor subunits. Mol Immunol 2006;43:1432-42. [Crossref] [PubMed]
- Wheeler DW, Thompson AJ, Corletto F, et al. Anaesthetic impairment of immune function is mediated via GABA(A) receptors. PLoS One 2011;6:e17152. [Crossref] [PubMed]
- Zhang B, Vogelzang A, Miyajima M, et al. B cell-derived GABA elicits IL-10(+) macrophages to limit anti-tumour immunity. Nature 2021;599:471-6. [Crossref] [PubMed]
- Dionisio L, José De Rosa M, Bouzat C, et al. An intrinsic GABAergic system in human lymphocytes. Neuropharmacology 2011;60:513-9. [Crossref] [PubMed]
- Mendu SK, Bhandage A, Jin Z, et al. Different subtypes of GABA-A receptors are expressed in human, mouse and rat T lymphocytes. PLoS One 2012;7:e42959. [Crossref] [PubMed]
- Bhat R, Axtell R, Mitra A, et al. Inhibitory role for GABA in autoimmune inflammation. Proc Natl Acad Sci U S A 2010;107:2580-5. [Crossref] [PubMed]
- Jin Z, Mendu SK, Birnir B. GABA is an effective immunomodulatory molecule. Amino Acids 2013;45:87-94. [Crossref] [PubMed]
- Reyes-García MG, Hernández-Hernández F, Hernández-Téllez B, et al. GABA (A) receptor subunits RNA expression in mice peritoneal macrophages modulate their IL-6/IL-12 production. J Neuroimmunol 2007;188:64-8. [Crossref] [PubMed]
- Tian J, Kaufman DL. The GABA and GABA-Receptor System in Inflammation, Anti-Tumor Immune Responses, and COVID-19. Biomedicines 2023;11:254. [Crossref] [PubMed]
- Olsen RW. GABA(A) receptor: Positive and negative allosteric modulators. Neuropharmacology 2018;136:10-22. [Crossref] [PubMed]
- Weir CJ, Mitchell SJ, Lambert JJ. Role of GABAA receptor subtypes in the behavioural effects of intravenous general anaesthetics. Br J Anaesth 2017;119:i167-75. [Crossref] [PubMed]
- Zhang T, Fan Y, Liu K, et al. Effects of different general anaesthetic techniques on immune responses in patients undergoing surgery for tongue cancer. Anaesth Intensive Care 2014;42:220-7. [Crossref] [PubMed]
- Lim JA, Oh CS, Yoon TG, et al. The effect of propofol and sevoflurane on cancer cell, natural killer cell, and cytotoxic T lymphocyte function in patients undergoing breast cancer surgery: an in vitro analysis. BMC Cancer 2018;18:159. [Crossref] [PubMed]
- Murray PJ, Allen JE, Biswas SK, et al. Macrophage activation and polarization: nomenclature and experimental guidelines. Immunity 2014;41:14-20. [Crossref] [PubMed]
- Lawrence T, Natoli G. Transcriptional regulation of macrophage polarization: enabling diversity with identity. Nat Rev Immunol 2011;11:750-61. [Crossref] [PubMed]
- Daigneault M, Preston JA, Marriott HM, et al. The identification of markers of macrophage differentiation in PMA-stimulated THP-1 cells and monocyte-derived macrophages. PLoS One 2010;5:e8668. [Crossref] [PubMed]
- Genin M, Clement F, Fattaccioli A, et al. M1 and M2 macrophages derived from THP-1 cells differentially modulate the response of cancer cells to etoposide. BMC Cancer 2015;15:577. [Crossref] [PubMed]
- Kochiyama T, Li X, Nakayama H, et al. Effect of Propofol on the Production of Inflammatory Cytokines by Human Polarized Macrophages. Mediators Inflamm 2019;2019:1919538. [Crossref] [PubMed]
- Sanders RD, Grover V, Goulding J, et al. Immune cell expression of GABAA receptors and the effects of diazepam on influenza infection. J Neuroimmunol 2015;282:97-103. [Crossref] [PubMed]
- Olsen RW, Sieghart W. GABA A receptors: subtypes provide diversity of function and pharmacology. Neuropharmacology 2009;56:141-8. [Crossref] [PubMed]
- Kim JJ, Gharpure A, Teng J, et al. Shared structural mechanisms of general anaesthetics and benzodiazepines. Nature 2020;585:303-8. [Crossref] [PubMed]
- Sanders RD, Godlee A, Fujimori T, et al. Benzodiazepine augmented γ-amino-butyric acid signaling increases mortality from pneumonia in mice. Crit Care Med 2013;41:1627-36. [Crossref] [PubMed]
- Obiora E, Hubbard R, Sanders RD, et al. The impact of benzodiazepines on occurrence of pneumonia and mortality from pneumonia: a nested case-control and survival analysis in a population-based cohort. Thorax 2013;68:163-70. [Crossref] [PubMed]
- Yocum GT, Turner DL, Danielsson J, et al. GABA(A) receptor α(4)-subunit knockout enhances lung inflammation and airway reactivity in a murine asthma model. Am J Physiol Lung Cell Mol Physiol 2017;313:L406-15. [Crossref] [PubMed]
- Seifi M, Rodaway S, Rudolph U, et al. GABA(A) Receptor Subtypes Regulate Stress-Induced Colon Inflammation in Mice. Gastroenterology 2018;155:852-864.e3. [Crossref] [PubMed]
- Li Y, Song D, Bo F, et al. Diazepam inhibited lipopolysaccharide (LPS)-induced pyroptotic cell death and alleviated pulmonary fibrosis in mice by specifically activating GABA(A) receptor α4-subunit. Biomed Pharmacother 2019;118:109239. [Crossref] [PubMed]
Cite this article as: Kochiyama T, Koyama S, Sugasawa Y, Yamaguchi A, Fukuda M, Kawagoe I. Modifications in gamma-aminobutyric acid type A receptor subunit gene expression after macrophage differentiation and propofol administration in THP-1 cells. AME Med J 2024;9:31.