Pathophysiology of metabolic changes and malnutrition in colorectal cancer’s patients
Introduction
Colorectal cancer (CRC) is the third most common cancer worldwide (1). Metabolic changes and malnutrition are a common feature in this group of patients, affecting treatments, outcomes, and quality of life (2-7). Chemo and radiotherapies, biologics, and surgical therapies, ultimately worse the abovementioned metabolic changes, increasing the risk of malnutrition (8,9).
Metabolic changes of CRC patients regard all metabolic pathways, including the metabolism of glucose, lipids, proteins, and nucleic acids. Glucose metabolism variations lead to hyperglycemia and/or insulin-resistance (IR) (10,11). Lipid metabolism impairment increases cholesterol, triglyceride, and free fatty acid (FFA) blood levels (12,13). Protein metabolism is shifted towards an increased protein degradation with the reduction of protein synthesis, due to increased nucleic acid degradation (14,15). All these changes reduce the amount of available energy for the physiologic cellular activity contributing to the inflammatory status typical of oncologic patients (16-19) and originating a vicious cycle that furtherly worsen the metabolic status, outcomes, and prognosis.
IR, a body adaptation response to situations of severe stress, such as in case of severe hemorrhages, leading to glucose mobilization, fluids shift and plasma refill, can ultimately impair outcomes. In fact, IR is an independent factor responsible of prolonging length of hospital stays, and hyperglycemia increases the risk of post-operative complications, such as surgical site infections (SSIs), and mortality (20-22).
Changes in proteins metabolism causes asthenia and sarcopenia and reduces strength; increase oncologic treatments’ complications rate or make it impossible to offer one (23,24). Together with lipids metabolism changes, these alterations lead to malnutrition, worsen patients’ prognosis, and ultimately bring patients to cancer-related cachexia. In this setting, it is particularly important to assess and re-equilibrate CRC patients metabolic and nutritional status before any therapeutic intervention is planned.
The present review summarizes causes and effects of metabolic changes affecting CRC patients undergoing medical and surgical treatments and offers an overview of clinical interventions to reduce these changes and improve patients’ outcome.
Metabolic changes in CRC patients
Changes in inflammatory signaling
Metabolic disorders, mostly leading to malnutrition, are extremely common and can be considered a multifactorial complication of each cancer. Cancer-related cachexia can be considered a form of end stage metabolic impairment; it might occur in half of all CRC patients and is responsible in up to one fifth of the cases of jeopardizing therapeutic options and, ultimately of cancer-related deaths (25).
The complex interaction between the tumor microenvironment and its host generates an inflammatory response affecting hunger and satiety and promoting tumor progression (26).
Inflammatory cytokines produced in CRC patients include tumor necrosis factor α (TNF-α), interleukin 6 (IL-6), calcitonin gene-related peptide (CGRP) and insulin-like growth factor 1 (IGF-1) (Figure 1).
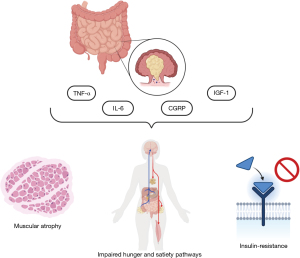
TNF-α has a catabolic action, but its blood levels are not directly associated with weight loss. It increases gluconeogenesis, glycogen synthesis and proteolysis, reduces proteins synthesis, and causes muscular atrophy (27). Its catabolic effect on skeletal muscle is also mediated by the activation of nuclear factor-kappa B (NF-κB) pathways (28,29). It activates the hypothalamus-pituitary-adrenocortical (HPA) axis (30), affecting metabolism through cortisol production and can interfere with intestinal nutrients absorption (31). Its action on metabolic changes seems to be mediated also by the alteration of leptin effects (32). Leptin is an anorexigenic peptide hormone, usually produced by adipocytes. It has a central action mediated by hypothalamic receptors, stimulating anorexigenic neuropeptides and inhibiting orexigenic neuropeptides production. Moreover, it affects thermogenesis, a homeostatic mechanism that usually limits weight gain in response to caloric excess. However, whether inflammatory cytokines reduce leptin production or impair its action is not clear, yet.
IL-6 is an inflammatory cytokine with pleiotropic effects, associated with increased CRC aggressivity and worse prognosis. Its concentration is correlated with serum-FFAs, both in early and late-stage CRC. This cytokine promotes muscular atrophy, with mechanisms mainly described in animal models, mostly based on the suppression of protein synthesis through Janus Kinase (JAK) pathways (33). Its production may also increase energy expenditure and lead to decreased food intake (34). The metabolic changes it causes are mainly due to leptin function impairment and HPA axis activation, which increases cortisol blood levels.
CGRP affects hungry and satiety pathways, reducing the former and increasing the latter, with mechanisms probably mediated by its interaction with peptide YY (PYY) receptor 2 in the hypothalamus (35). It seems CGRP could also affect the production of neuropeptide Y and other molecules involved in hunger and satiety pathways.
IGF-1 is a peptide hormone usually produced by liver, adipocytes, muscle, and brain. Its anorexigenic action is mediated by the increased production of others anorexigenic molecules such as CGRP, PYY, etc. and the reduction in ghrelin blood levels, which is an orexigenic hormone usually produced by the stomach in case of fasting. Furthermore, IGF-1 directly interferes with brain insulin receptors (36). Many studies suggest IGF-1 and its receptor may also be involved in CRC pathogenesis. Indeed, IGF-1 can promote tumor growth stimulating cellular proliferation and survival through the interaction with its receptor or the pathway PI3K/AKT/mTOR, which is involved in protein synthesis and cellular homeostasis. Its blood levels are associated to increased cancer aggressivity (15,37).
From a clinical point of view, these inflammatory and metabolic changes lead to a remarkably different state of starvation with respect to starvation-associated weight loss, with loss of lean mass and skeletal muscle more than adipose tissue (38).
Clinical changes
Appetite loss in CRC patients can be caused by tumor volume, leading to bowel stenosis causing obstruction, a condition associated with poor oncologic prognosis (39,40). CRC can also alter the mucosal barrier, characterized by the disruption and leakage of the intestinal epithelium, which leads to translocation into the circulation of both bacteria and their components, contributing to the general inflammatory status, participating to the above-mentioned metabolic changes cascade.
Changes in intestinal microbiota
Gut barrier dysfunction also impairs nutrients absorption, worsening the energy imbalance typical of CRC patients (41,42). Furthermore, CRC patients have shown reduced microbiota diversity and richness compared with healthy individuals (43). These patients present a reduction in probiotics bacteria and increased enterobacteria concentration. The formers include butyrate-producers as Clostridium butyicum, that produce short-chain fatty acids with anti-inflammatory effects and may exert a protective function against CRC. On the contrary, enterobacteria such as Fusobacterium nucleatum and Enterococcus faecalis seems to contribute to disease progression from adenoma to cancer and indicated a worse prognosis, probably because of superoxide production, which damages DNA in epithelial cells (44). Beside the detrimental actions of enterobacteria surpassing those of the beneficial commensals, microbiota changes alter intestinal mucosa integrity, impairing its permeability to bacteria and toxic substances, but also affecting nutrients absorption and activating the HPA axis (45).
Metabolic changes induced by neo-adjuvant or adjuvant treatments
Cytotoxic therapy
Beyond cancer-specific metabolic impairment, chemotherapy (CT), radiotherapy (RT) and biologics, further participate to metabolic compromission, eventually contributing to malnutrition and cachexia (Table 1).
Table 1
Drug family | Drug | Gastrointestinal side effects | Pathophysiology |
---|---|---|---|
Fluoropyrimidine | Fluorouracil | • Dose-related diarrhea | Interruption of mitosis of intestinal crypt cells |
• Malabsorption | |||
Capecitabine | • Dose-related diarrhea | ||
• Malabsorption | |||
Camptothecins | Irinotecan | • Diarrhea | SN38 causes: |
• Direct mucosal damage | |||
• Luminal environment alteration | |||
• Increased mucin secretion | |||
• Villous atrophy | |||
Platinum | Cisplatin | • Nausea and vomiting | • 5-HT release induces nausea and vomiting |
• Weight loss | • NF-κB pathway causes muscular wasting | ||
• Diarrhea | • Reduced ghrelin production impairs hunger and satiety pathways | ||
• Mucositis and stomatitis | |||
• Anorexia | |||
• Muscular wasting | |||
Biologics | EGFR | • Malabsorption | • Damaged enterocyte transporters impair electrolytes flux |
• Diarrhea (severe in up to 30% of patients) |
• Inhibition of E-cadherin production affects mucosal integrity and permeability | ||
• Intestinal mobility is increased |
SN38, 7-ethyl-10-hydroxycamptothecin; 5-HT, 5-hydroxytryptamine; NF-κB, nuclear factor-kappa B; EGFR, epidermal growth factor receptor.
CRC medical treatment is mostly based on the administration of fluoropyrimidine, either 5-fluorouracil (FU) or capecitabine, combined with irinotecan or platinum (46,47). Biologics include monoclonal antibodies or proteins against the vascular endothelial growth factor (VEGF) and the epidermal growth factor receptor (EGFR). Their administration in combination with CT is restricted to metastatic disease (27). RT is used as local treatment for rectal cancer (both in the neoadjuvant and adjuvant setting) (48) and occasionally for locally advanced colon cancer.
Fluoropyrimidines show gastrointestinal toxicity causing dose-related diarrhea, and electrolytes imbalance. When leucovorin is added, the percentage of patients with severe diarrhea rise to 50% (49). The pathophysiology of fluoropyrimidine-induced diarrhea has not been deeply investigated, yet. It seems that fluoropyrimidines cause the interruption of mitosis of intestinal crypt cells, decreasing the resorption surface area leading both to diarrhea and malabsorption (50). Capecitabine shows reduced gastrointestinal toxicity compared to FU (51) whilst irinotecan can cause diarrhea, too. Despite the detailed mechanisms leading to diarrhea are still a matter of debate, it seems they can be attributable to its active metabolite 7-ethyl-10-hydroxycamptothecin (SN38), which is produced in the intestinal lumen by bacterial ß-glucuronidase. Free intestinal SN38 could induce direct mucosal damage, could alter luminal environment favoring proliferation of different bacteria, could increase mucin secretion and/or could cause villous atrophy impairing absorption and causing diarrhea (49).
Cancer platinum-based treatment can affect metabolism causing weight loss, nausea and vomiting, diarrhea, mucositis, stomatitis, anorexia, cachexia, and inflammation. The most common gastrointestinal side effects are caused by 5-hydroxytryptamine (5-HT) released by intestinal cells target of platinum drugs and binding serotonin receptors both in central and peripheral nervous system, thus leading to nausea and vomiting. Cisplatin has the highest rates of nausea and vomiting, which are shown in up to 90% of patients (52). Moreover, it can regulate muscular wasting through the activation of NF-κB pathway (25) and seems to reduce ghrelin production, impairing hunger and satiety pathways (53).
In general, all cytotoxic drugs, share a range of side effects due to their poor selectivity for cancer cells. These drugs are taken up by all fast-reproducing cells, including cancer cells. However, they can affect also fast regenerating tissues such as the mucous membranes of the mouth, stomach, and intestines, causing gastrointestinal toxicity (meaning weight loss, nausea and vomiting, diarrhea, mucositis, stomatitis, and malabsorption) (52). Finally, CT stimulates proteolysis and proinflammatory cytokines production such as TNF-α and IL-6 (54,55), thus indirectly affecting metabolic pathways.
Recent literature focused on the impact CT has on gut microbiota (56-58). Its action is mediated by proinflammatory cytokines increasing mucin secretion. Mucin reduces adhesion sites and affects luminal nutrients availability, inhibiting Lactobacilli and Bifidobacteria proliferation and stimulating Clostridium and E. coli (59). Every imbalance of intestinal microbiota can determine epithelial intestinal mucosa damage or luminal variation in terms of digestive enzymes balance, leading to malabsorption and translocation of bacteria or toxins.
Biological therapies
Biological therapies to treat cancer are derived from living organisms that can modify the immune response, but they can alter patients’ metabolism, too. Anti-EGFR drugs can cause malabsorption and severe diarrhea in up to 30% of patients. The multiple mechanisms leading to gastrointestinal toxicity include impaired electrolytes flux across the enterocyte membrane due to damaged transporters, the presence of hypertonic substances in the intestinal lumen, and increased gut motility (60). Moreover, anti-EGFR drugs inhibit E-cadherin production, reducing mucosal integrity and permeability (61). This process affects nutrients and toxins absorption, leading to an inflammatory general status which contributes to metabolic changes.
RT
RT effects on patient’s metabolism are mainly due to mucositis causing nausea, vomit, diarrhea, malabsorption, and pain, depending on the area of exposure. Both acute and delayed RT-induced gastrointestinal toxicities are caused by intestinal epithelium injury. This is mediated by NF-κB, mitogen-activated protein kinase (MAPK), and matrix metalloproteinases (MMP) in the acute setting, meaning shortly after RT administration and up to 3 months later. Delayed impairment, showed between 18 months and 6 years after RT administration, is mediated by transforming growth factor-b1 (TGF-b1) and thrombomodulin, that alter extracellular matrix (ECM) (62).
Metabolic changes induced by CRC surgery
Surgical stress
Most CRC patients will eventually undergo surgery, which plays a key role in the treatment of both localized disease and in selected advanced metastatic patients (39,40,46-48). It means, CRC patients mostly face metabolic alterations caused by surgery.
It is well known that every injury, thus also an operation, determine a stress response characterized by an inflammatory condition inducing a catabolic state (63). Indeed, inflammatory mediators and stress hormones production leads to catabolism of glycogen, fat, and proteins, reducing peripheral protein mass, especially muscles. Release of glucose, FFA, and amino acids from the body, aims to improve the immune response and healing.
Following surgical trauma, the stress response is activated. The nervous system sends impulses from the injured site to the hypothalamus, which modifies pituitary hormones release and/or production. Inflammatory cytokines also play a key role increasing pituitary hormones secretion. The most involved hormones in metabolic changes are corticotrophin (ACTH) and growth hormone (GH), both acting on their own target organs. This cascade of events leads to increased blood levels of cortisol, glucagon, catecholamines, together with proinflammatory molecules (64). As a result of hormonal changes, peripheral response to insulin release decreases [insulin resistance (IR)], for over than a week after the operation, proportionally to its magnitude (65,66). After a colorectal operation, up to 90% of the preoperative insulin sensitivity can be lost (65). Such a modification in insulin peripheral sensitivity can last up to 2–4 weeks after uncomplicated surgery. This is the central mechanism to achieve the catabolic state. IR causes a compensatory rise in insulin production, leading to the release of glucose from the hepatic stores of glycogen. Decreased peripheral glucose utilization worsens hyperglycemia. Moreover, the post-operative catabolic state resulting from IR, is also characterized by an increased protein breakdown, involving both albumin and other proteins.
Surgery and intestinal microbiota
Colorectal surgery also affects gut microbiota composition, thus indirectly affecting absorption and causing metabolic changes. Microbiota composition during and after surgery is altered because of several factors including stress, the alteration of body homeostasis, exposure of the intestinal lumen to oxygen, and tissue ischemia. All these factors determine a shift in the composition of the microbiota localized both on the intestinal mucosa and in its lumen, with a reduction of the so-called good anaerobes species and a gain of bad facultative aerobes species, as enterococcus. Changes in mucosal-associated microbiota are associated to altered mucin secretion and short-chain fatty acids production, leading to impaired nutrient absorption, damage of the intestinal barrier, bacterial translocation and a general proinflammatory state that contributes to the metabolic changes induced by surgery (67).
Surgical resections
Colorectal resections are known to participate in malabsorption. For transverse and left colon and for rectal cancer patients, the resection is only minimally responsible, even though for anterior resections, a well-known syndrome, the low anterior resection syndrome (LARS), certainly affect nutritional status (68). On the contrary, patients undergoing right hemicolectomy will lose their ileo-cecal valve and terminal ileum, thus biliary acid and microelements.
Perioperative measures
Metabolic changes induced by the surgical operation itself can be furtherly affected by the measures adopted in the perioperative period. Indeed, despite the large amount of evidence supporting reduced perioperative fasting, still many patients face overnight fasting before surgery and long post-operative restriction both in food and oral fluids intake. Tolerance to normal food or enteral nutrition after surgery can be affected by post-operative ileus, which may be exacerbated by laparotomic procedures, intraoperative manipulation and subsequent pan enteric inflammation, excessive intravenous fluid load, both during and after the operation causing intestinal wall oedema, opioids administration, prolonged bed rest (69).
Despite the different sources of metabolic alterations in CRC patients undergoing surgery, recent literature is mostly focusing on the peri-operative stress, seeking for strategies which could minimize catabolism and support anabolism, thus accelerating the post-operative recovery.
Improving metabolic status: a team effort
As previously highlighted, metabolic changes affect outcomes of CRC patient’s treatment. Recognizing and managing them properly is crucial to increasing the chance to complete every step of the scheduled anticancer therapies, and to optimize treatments outcomes including overall survival and quality of life. For this reason, a multidisciplinary approach is required to optimize patients’ metabolic status, maintaining skeletal mass and physical performances. Several professional figures are involved in the process, including nurses, nutritionists, physiotherapists, psychologists, oncologists, gastroenterologists, and surgeons. The whole team works on what is known as a pre-habilitation program. Counselling on nutritional support is offered by nurses and nutritionists. The management of cancer-associated malnutrition includes nutritional counselling informing patients about nutritional topics and showing them healthy eating habits. Moreover, cancer patients frequently need nutritional supplements, nutrient mixtures orally administered if volitional food intake does not guarantee the complete metabolic recovery. If nutrient intake remains inadequate, supplemental nutrition by the enteral or parenteral route may be indicated (70). Physiotherapists should offer and adapt to single needs a correct physical program necessary to gain or re-gain physical strength. If needed a psycho-oncologist will provide the necessary support, whilst oncologists and gastroenterologists will deal with medical therapies to reduce the most detrimental side effects of the oncologic treatment, such as antiemetics, analgesics, drugs increasing or reducing salivary production depending on the specific case, proton pump inhibitors to reduce gastritis or esophagitis. Surgeons and anesthesiologist will apply all the necessary measures to reduce surgical stress, such as programs of enhance recovery and perioperative optimization.
For what concern the management of the metabolic alterations induced by CRC treatments, the literature offers several evidences.
Management of medical treatment-induced alterations
One of the principal efforts is focused on the management of CT-induced diarrhea, which is based on both nonpharmacological and pharmacological strategies. The formers are based on dietary advice, avoiding aliments that worsen numbers and severity of liquid stools, increasing fluids and salts to keep patient hydrated. If the nonpharmacological approach is not sufficient, there are several drugs which have been proposed to treat diarrhea. Budesonide, glutamine, celecoxib, long-acting octreotide, as well as marginally absorbable antibiotics like neomycin, all seems to be of some efficacy in treating CT-induced diarrhea even though, only loperamide and octreotide are suggested by guidelines (49). Loperamide is an opioid which alters the function of the intestinal mucosa smooth muscle and reduces bowel movement frequency and stool weight. Octreotide is a synthetic somatostatin analog, which is used only as second line treatment in case of refractory-to-loperamide diarrhea. Its action is mediated by the reduction of vasoactive intestinal peptide (VIP) blood levels, that slows down intestinal transit, increases fluid absorption, and reduces mucosal secretions (49). Both can be used for the treatment of diarrhea induced by both chemotherapeutic agents and biological therapies.
Platinum-induced nausea and vomiting is another frequent CT side effect. Treatment is based on the administration of a combination of antiemetics, depending on the used platinum regimen. Cisplatin and carboplatin cause more intense gastrointestinal side effects, thus their treatment requires a combination of at least 3–4 drugs including 5-HT antagonists, neurokinin 1 (NK1) antagonists, dexamethasone, and olanzapine. Oxaliplatin side effects are less intense, thus dexamethasone administration is usually sufficient.
Management of surgery-induced alterations
In elective surgery it has been shown that the best way to minimize catabolism and support anabolism is to reduce post-operative stress through perioperative programs collected in enhanced recovery protocols (71-73). The most important items affecting metabolism are the reduction of fasting, preoperative carbohydrate load and early post-operative feeding, avoiding long starvation periods. However, also post-operative nausea and vomiting (PONV) control, pain control, application of standard anesthesia protocols and intra-operative fluid restriction have a role in surgical stress reduction and affect hunger and satiety pathways. The combination of all these treatments can minimize IR, allowing full glucose control without insulin administration even in patients who underwent major colorectal surgery and started early enteral nutrition (74).
The most discussed items are the appropriate pre-operative carbohydrate loading and the length of pre-operative fasting period. Recent evidence recommends reduction of pre-operative fasting, allowing solid assumption up to 6 hours and clear fluids up to 2 hours before surgery (71). These novel protocols improve subjective well-being, reducing thirst, dryness of mouth and anxiety (75).
Pre-operative carbohydrate load reduces post-operative IR, too. Moreover, it can improve thirst and reduce protein loss, easily assessed with muscular strength. In elective colorectal surgery 285 mOsm/kg of complex maltodextrin administration before surgery is suggested: diluted in 800 mL of water to be taken the evening before surgery and in 400 mL of water to drink 2–3 h before the induction of anesthesia (71). Despite the good impact on post-operative metabolic status, carbohydrate load cannot be administered to diabetic patients because of the excessive increase in glycemic values. Its administration is contraindicated also in patients affected by gastroesophageal reflux disease (GERD) or delayed gastric emptying; a large amount of carbohydrates can further slowdown gastric emptying, increasing the risk of aspiration in patients with impaired gastric function.
Immunonutrition also seems to influence host immune response to surgical stress (76-78). It is based on the administration of immune-modulating nutrients in higher doses than standard nutritional protocols. Further evidence is necessary to establish their exact role.
Laparoscopic surgery and, in general, minimally invasive surgery, also seems to improve glucose metabolism compared to open surgery, providing faster recovery of nutritional and immunologic status (31,79-81).
Whether perioperative management or minimally invasive surgery have a major impact on metabolic changes is not clear, yet. Xu et al. compared their effect on the nutritional status of CRC patients through albumin, prealbumin and transferrin levels assessment. The analysis of blood samples collected preoperatively, at 12 and 96 hours after surgery, showed accelerate rising in albumin levels in patients undergoing laparoscopic surgery, regardless of fats-track treatment highlighting both a direct and indirect beneficial effect of laparoscopy on patients’ metabolism (79). However, more recent studies suggest an indirect relation between the surgical technique and metabolic changes, due to the reduction of post-operative inflammation (82,83). Indeed, Siekmann et al. assessed cortisol, insulin and serum cytokines levels in blood samples collected preoperatively, 1–6 hours postoperatively and 3–5 days postoperatively. The study did not show any difference in plasma cortisol and insulin levels between groups. On the contrary, higher cytokines levels were found few hours after the procedure in patients undergoing open surgery rather than laparoscopy (84), indirectly affecting IR and metabolism in general.
The reduction of post-operative inflammation is not the only beneficial effect of laparoscopic surgery. Pędziwiatr et al. showed laparoscopy can also reduce the negative impact of sarcopenia (a result of malnutrition) on post-operative outcomes (85). Sarcopenia is a depletion of skeletal muscle mass and strength, usually associated to myosteatosis (fat infiltration in skeletal muscle) (86). It is the result of the state of chronic inflammation associated to IR and nutritional impairment (87) and is a well-known risk factor for post-operative infections and delayed recovery after traditional CRC surgery (88). Recent literature is focusing on the impact that laparoscopic surgery has on sarcopenic CRC patients, showing that laparoscopy can improve post-operative outcomes, significantly reducing overall complications rate, length of hospital stays, and readmission rate (85,89). The relationship between sarcopenia and laparoscopy needs to be investigated further, but it could be speculated that improving nutritional status before surgery could possibly improve the already excellent results of minimally invasive surgery.
Conclusions
This review summarizes mechanisms leading to metabolic changes and malnutrition in CRC patients undergoing treatment. There is an unequivocal risk that metabolic changes and malnutrition jeopardize cancer treatment and ultimately impairs clinical outcomes and cancer related survival. Unfortunately, not all the mechanisms leading to metabolic changes are completely understood. Some of them have been described only in animal models and others, like fluoropyrimidine- and irinotecan-induced diarrhea, are still unclear. Both, medical and surgical measures adopted in the perioperative treatment period are mostly symptoms bonded. Furthermore, most of the in-use protocols are still under discussion because, despite the many positive results, they often lack proper evidence. Further studies investigating metabolic changes in CRC patients will certainly help to reach a deeper understanding, allowing to increase available therapeutic means.
Acknowledgments
Funding: None.
Footnote
Peer Review File: Available at https://amj.amegroups.com/article/view/10.21037/amj-23-154/prf
Conflicts of Interest: All authors have completed the ICMJE uniform disclosure form (available at https://amj.amegroups.com/article/view/10.21037/amj-23-154/coif). The authors have no conflicts of interest to declare.
Ethical Statement: The authors are accountable for all aspects of the work in ensuring that questions related to the accuracy or integrity of any part of the work are appropriately investigated and resolved.
Open Access Statement: This is an Open Access article distributed in accordance with the Creative Commons Attribution-NonCommercial-NoDerivs 4.0 International License (CC BY-NC-ND 4.0), which permits the non-commercial replication and distribution of the article with the strict proviso that no changes or edits are made and the original work is properly cited (including links to both the formal publication through the relevant DOI and the license). See: https://creativecommons.org/licenses/by-nc-nd/4.0/.
References
- WHO. International Agency for Research on Cancer. Available online: https://gco.iarc.fr/today/online-analysis-pie?v=2020&mode=cancer&mode_population=continents&population=900&populations=900&key=total&sex=0&cancer=39&type=0&statistic=5&prevalence=0&population_group=0&ages_group%5B%5D=0&ages_group%5B%5D=17&nb_items=7&grou
- Choi MH, Oh SN, Lee IK, et al. Sarcopenia is negatively associated with long-term outcomes in locally advanced rectal cancer. J Cachexia Sarcopenia Muscle 2018;9:53-9. [Crossref] [PubMed]
- Gannavarapu BS, Lau SKM, Carter K, et al. Prevalence and Survival Impact of Pretreatment Cancer-Associated Weight Loss: A Tool for Guiding Early Palliative Care. J Oncol Pract 2018;14:e238-50. [Crossref] [PubMed]
- Karabulut S, Dogan I, Usul Afsar C, et al. Does nutritional status affect treatment tolarability, response and survival in metastatic colorectal cancer patients? Results of a prospective multicenter study. J Oncol Pharm Pract 2021;27:1357-63. [Crossref] [PubMed]
- Sica GS. Guest edited collection: current friends and foes in gastrointestinal cancer. Discov Oncol 2021;12:34. [Crossref] [PubMed]
- Muscaritoli M, Lucia S, Farcomeni A, et al. Prevalence of malnutrition in patients at first medical oncology visit: the PreMiO study. Oncotarget 2017;8:79884-96. [Crossref] [PubMed]
- Cao X, Zhao G, Yu T, et al. Preoperative Prognostic Nutritional Index Correlates with Severe Complications and Poor Survival in Patients with Colorectal Cancer Undergoing Curative Laparoscopic Surgery: A Retrospective Study in a Single Chinese Institution. Nutr Cancer 2017;69:454-63. [Crossref] [PubMed]
- Gallois C, Bourillon C, Auclin E, et al. Skeletal muscle loss during chemotherapy and its association with survival and systemic treatment toxicity in metastatic colorectal cancer: An AGEO prospective multicenter study. Clin Res Hepatol Gastroenterol 2021;45:101603. [Crossref] [PubMed]
- van Rooijen S, Carli F, Dalton SO, et al. Preoperative modifiable risk factors in colorectal surgery: an observational cohort study identifying the possible value of prehabilitation. Acta Oncol 2017;56:329-34. [Crossref] [PubMed]
- Kasprzak A. Insulin-Like Growth Factor 1 (IGF-1) Signaling in Glucose Metabolism in Colorectal Cancer. Int J Mol Sci 2021;22:6434. [Crossref] [PubMed]
- Bellia A, Rizza S, Lombardo MF, et al. Deterioration of glucose homeostasis in type 2 diabetic patients one year after beginning of statins therapy. Atherosclerosis 2012;223:197-203. [Crossref] [PubMed]
- Pakiet A, Kobiela J, Stepnowski P, et al. Changes in lipids composition and metabolism in colorectal cancer: a review. Lipids Health Dis 2019;18:29. [Crossref] [PubMed]
- Bellia A, Rizza S, Galli A, et al. Early vascular and metabolic effects of rosuvastatin compared with simvastatin in patients with type 2 diabetes. Atherosclerosis 2010;210:199-201. [Crossref] [PubMed]
- Tisdale MJ. Mechanisms of cancer cachexia. Physiol Rev 2009;89:381-410. [Crossref] [PubMed]
- Argilés JM, Busquets S, Stemmler B, et al. Cancer cachexia: understanding the molecular basis. Nat Rev Cancer 2014;14:754-62. [Crossref] [PubMed]
- Nakamura Y, Imada A, Fukugaki A, et al. Association of nutritional risk and systemic inflammation with survival in patients with colorectal cancer who underwent curative surgery. Clin Nutr ESPEN 2022;49:417-24. [Crossref] [PubMed]
- Yang M, Lin SQ, Liu XY, et al. Association between C-reactive protein-albumin-lymphocyte (CALLY) index and overall survival in patients with colorectal cancer: From the investigation on nutrition status and clinical outcome of common cancers study. Front Immunol 2023;14:1131496. [Crossref] [PubMed]
- Ishizuka M, Nagata H, Takagi K, et al. Inflammation-based prognostic score is a novel predictor of postoperative outcome in patients with colorectal cancer. Ann Surg 2007;246:1047-51. [Crossref] [PubMed]
- Amelio I, Bertolo R, Bove P, et al. Cancer predictive studies. Biol Direct 2020;15:18. [Crossref] [PubMed]
- Thorell A, Nygren J, Ljungqvist O. Insulin resistance: a marker of surgical stress. Curr Opin Clin Nutr Metab Care 1999;2:69-78. [Crossref] [PubMed]
- Shariq OA, Hanson KT, McKenna NP, et al. Does Metabolic Syndrome Increase the Risk of Postoperative Complications in Patients Undergoing Colorectal Cancer Surgery? Dis Colon Rectum 2019;62:849-58. [Crossref] [PubMed]
- Zarzavadjian Le Bian A, Denet C, Tabchouri N, et al. The effect of metabolic syndrome on postoperative outcomes following laparoscopic colectomy. Tech Coloproctol 2018;22:215-21. [Crossref] [PubMed]
- Sierra JC, Dock-Nascimento DB, Behne TEG, et al. Major oncological surgery reduces muscular function in patients with or without nutritional risk. Rev Col Bras Cir 2020;47:e20202470. [Crossref] [PubMed]
- Schinzari F, Iantorno M, Campia U, et al. Vasodilator responses and endothelin-dependent vasoconstriction in metabolically healthy obesity and the metabolic syndrome. Am J Physiol Endocrinol Metab 2015;309:E787-92. [Crossref] [PubMed]
- Peixoto da Silva S, Santos JMO, Costa E. Cancer cachexia and its pathophysiology: links with sarcopenia, anorexia and asthenia. J Cachexia Sarcopenia Muscle 2020;11:619-35. [Crossref] [PubMed]
- Kasprzak A. The Role of Tumor Microenvironment Cells in Colorectal Cancer (CRC) Cachexia. Int J Mol Sci 2021;22:1565. [Crossref] [PubMed]
- Tisdale MJ. Catabolic mediators of cancer cachexia. Curr Opin Support Palliat Care 2008;2:256-61. [Crossref] [PubMed]
- Patel HJ, Patel BM. TNF-α and cancer cachexia: Molecular insights and clinical implications. Life Sci 2017;170:56-63. [Crossref] [PubMed]
- Han Y, Weinman S, Boldogh I, et al. Tumor necrosis factor-alpha-inducible IkappaBalpha proteolysis mediated by cytosolic m-calpain. A mechanism parallel to the ubiquitin-proteasome pathway for nuclear factor-kappab activation. J Biol Chem 1999;274:787-94. [Crossref] [PubMed]
- Beishuizen A, Thijs LG. Endotoxin and the hypothalamo-pituitary-adrenal (HPA) axis. J Endotoxin Res 2003;9:3-24. [PubMed]
- Fina D, Franzè E, Rovedatti L, et al. Interleukin-25 production is differently regulated by TNF-α and TGF-β1 in the human gut. Mucosal Immunol 2011;4:239-44. [Crossref] [PubMed]
- Coppack SW. Pro-inflammatory cytokines and adipose tissue. Proc Nutr Soc 2001;60:349-56. [Crossref] [PubMed]
- Muñoz-Cánoves P, Scheele C, Pedersen BK, et al. Interleukin-6 myokine signaling in skeletal muscle: a double-edged sword? FEBS J 2013;280:4131-48. [Crossref] [PubMed]
- Bonetto A, Aydogdu T, Jin X, et al. JAK/STAT3 pathway inhibition blocks skeletal muscle wasting downstream of IL-6 and in experimental cancer cachexia. Am J Physiol Endocrinol Metab 2012;303:E410-21. [Crossref] [PubMed]
- Russell FA, King R, Smillie SJ, et al. Calcitonin gene-related peptide: physiology and pathophysiology. Physiol Rev 2014;94:1099-142. [Crossref] [PubMed]
- Yu H, Rohan T. Role of the insulin-like growth factor family in cancer development and progression. J Natl Cancer Inst 2000;92:1472-89. [Crossref] [PubMed]
- Pollak M. Insulin-like growth factor physiology and cancer risk. Eur J Cancer 2000;36:1224-8. [Crossref] [PubMed]
- Porporato PE. Understanding cachexia as a cancer metabolism syndrome. Oncogenesis 2016;5:e200. [Crossref] [PubMed]
- Salvatore L, Imperatori M, Arnoldi E, et al. Management of patients with early-stage colon cancer: guidelines of the Italian Medical Oncology Association. ESMO Open 2020;5:e001001. [Crossref] [PubMed]
- Labianca R, Nordlinger B, Beretta GD, et al. Early colon cancer: ESMO Clinical Practice Guidelines for diagnosis, treatment and follow-up. Ann Oncol 2013;24:vi64-72. [Crossref] [PubMed]
- Klein GL, Petschow BW, Shaw AL, et al. Gut barrier dysfunction and microbial translocation in cancer cachexia: a new therapeutic target. Curr Opin Support Palliat Care 2013;7:361-7. [Crossref] [PubMed]
- Puppa MJ, White JP, Sato S, et al. Gut barrier dysfunction in the Apc(Min/+) mouse model of colon cancer cachexia. Biochim Biophys Acta 2011;1812:1601-6. [Crossref] [PubMed]
- Chen W, Liu F, Ling Z, et al. Human intestinal lumen and mucosa-associated microbiota in patients with colorectal cancer. PLoS One 2012;7:e39743. [Crossref] [PubMed]
- Cheng Y, Ling Z, Li L. The Intestinal Microbiota and Colorectal Cancer. Front Immunol 2020;11:615056. [Crossref] [PubMed]
- Frankiensztajn LM, Elliott E, Koren O. The microbiota and the hypothalamus-pituitary-adrenocortical (HPA) axis, implications for anxiety and stress disorders. Curr Opin Neurobiol 2020;62:76-82. [Crossref] [PubMed]
- Argilés G, Tabernero J, Labianca R, et al. Localised colon cancer: ESMO Clinical Practice Guidelines for diagnosis, treatment and follow-up. Ann Oncol 2020;31:1291-305. [Crossref] [PubMed]
- Cervantes A, Adam R, Roselló S, et al. Metastatic colorectal cancer: ESMO Clinical Practice Guideline for diagnosis, treatment and follow-up. Ann Oncol 2023;34:10-32. [Crossref] [PubMed]
- Glynne-Jones R, Wyrwicz L, Tiret E, et al. Rectal cancer: ESMO Clinical Practice Guidelines for diagnosis, treatment and follow-up. Ann Oncol 2018;29:iv263. [Crossref] [PubMed]
- Stein A, Voigt W, Jordan K. Chemotherapy-induced diarrhea: pathophysiology, frequency and guideline-based management. Ther Adv Med Oncol 2010;2:51-63. [Crossref] [PubMed]
- Siber GR, Mayer RJ, Levin MJ. Increased gastrointestinal absorption of large molecules in patients after 5-fluorouracil therapy for metastatic colon carcinoma. Cancer Res 1980;40:3430-6. [PubMed]
- Saif MW, Katirtzoglou NA, Syrigos KN. Capecitabine: an overview of the side effects and their management. Anticancer Drugs 2008;19:447-64. [Crossref] [PubMed]
- Oun R, Moussa YE, Wheate NJ. The side effects of platinum-based chemotherapy drugs: a review for chemists. Dalton Trans 2018;47:6645-53. [Crossref] [PubMed]
- Hattori T, Yakabi K, Takeda H. Cisplatin-induced anorexia and ghrelin. Vitam Horm 2013;92:301-17. [Crossref] [PubMed]
- Arrieta O, Michel Ortega RM, Villanueva-Rodríguez G, et al. Association of nutritional status and serum albumin levels with development of toxicity in patients with advanced non-small cell lung cancer treated with paclitaxel-cisplatin chemotherapy: a prospective study. BMC Cancer 2010;10:50. [Crossref] [PubMed]
- Ciorba MA, Riehl TE, Rao MS, et al. Lactobacillus probiotic protects intestinal epithelium from radiation injury in a TLR-2/cyclo-oxygenase-2-dependent manner. Gut 2012;61:829-38. [Crossref] [PubMed]
- Lin XB, Dieleman LA, Ketabi A, et al. Irinotecan (CPT-11) chemotherapy alters intestinal microbiota in tumour bearing rats. PLoS One 2012;7:e39764. [Crossref] [PubMed]
- Touchefeu Y, Montassier E, Nieman K, et al. Systematic review: the role of the gut microbiota in chemotherapy- or radiation-induced gastrointestinal mucositis - current evidence and potential clinical applications. Aliment Pharmacol Ther 2014;40:409-21. [Crossref] [PubMed]
- Lin XB, Farhangfar A, Valcheva R, et al. The role of intestinal microbiota in development of irinotecan toxicity and in toxicity reduction through dietary fibres in rats. PLoS One 2014;9:e83644. [Crossref] [PubMed]
- Liu L, Bai Y, Xiang L, et al. Interaction between gut microbiota and tumour chemotherapy. Clin Transl Oncol 2022;24:2330-41. [Crossref] [PubMed]
- Tao G, Chityala PK. Epidermal growth factor receptor inhibitor-induced diarrhea: clinical incidence, toxicological mechanism, and management. Toxicol Res (Camb) 2021;10:476-86. [Crossref] [PubMed]
- Fan L, Hu L, Yang B, et al. Erlotinib promotes endoplasmic reticulum stress-mediated injury in the intestinal epithelium. Toxicol Appl Pharmacol 2014;278:45-52. [Crossref] [PubMed]
- Stansborough RL, Al-Dasooqi N, Bateman EH, et al. Radiotherapy-induced gut toxicity: Involvement of matrix metalloproteinases and the intestinal microvasculature. Int J Radiat Biol 2016;92:241-8. [Crossref] [PubMed]
- Onali S, Calabrese E, Petruzziello C, et al. Post-operative recurrence of Crohn’s disease: A prospective study at 5 years. Dig Liver Dis 2016;48:489-94. [Crossref] [PubMed]
- Finnerty CC, Mabvuure NT, Ali A, et al. The surgically induced stress response. JPEN J Parenter Enteral Nutr 2013;37:21S-9S. [Crossref] [PubMed]
- Ljungqvist O. Insulin resistance and outcomes in surgery. J Clin Endocrinol Metab 2010;95:4217-9. [Crossref] [PubMed]
- Schricker T, Lattermann R. Perioperative catabolism. Can J Anaesth 2015;62:182-93. [Crossref] [PubMed]
- Agnes A, Puccioni C, D’Ugo D, et al. The gut microbiota and colorectal surgery outcomes: facts or hype? A narrative review. BMC Surg 2021;21:83. [Crossref] [PubMed]
- Siragusa L, Sensi B, Vinci D, et al. Volume-outcome relationship in rectal cancer surgery. Discov Oncol 2021;12:11. [Crossref] [PubMed]
- Weimann A, Braga M, Carli F, et al. ESPEN guideline: Clinical nutrition in surgery. Clin Nutr 2017;36:623-50. [Crossref] [PubMed]
- Arends J, Bachmann P, Baracos V, et al. ESPEN guidelines on nutrition in cancer patients. Clin Nutr 2017;36:11-48. [Crossref] [PubMed]
- Gustafsson UO, Scott MJ, Hubner M, et al. Guidelines for Perioperative Care in Elective Colorectal Surgery: Enhanced Recovery After Surgery (ERAS(®)) Society Recommendations: 2018. World J Surg 2019;43:659-95. [Crossref] [PubMed]
- Bellato V, An Y, Cerbo D, et al. Feasibility and outcomes of ERAS protocol in elective cT4 colorectal cancer patients: results from a single-center retrospective cohort study. World J Surg Oncol 2021;19:196. [Crossref] [PubMed]
- Sica GS, Campanelli M, Bellato V, et al. Gastrointestinal cancer surgery and enhanced recovery after surgery (ERAS) during COVID-19 outbreak. Langenbecks Arch Surg 2020;405:357-8. [Crossref] [PubMed]
- Soop M, Carlson GL, Hopkinson J, et al. Randomized clinical trial of the effects of immediate enteral nutrition on metabolic responses to major colorectal surgery in an enhanced recovery protocol. Br J Surg 2004;91:1138-45. [Crossref] [PubMed]
- Weimann A, Braga M, Carli F, et al. ESPEN practical guideline: Clinical nutrition in surgery. Clin Nutr 2021;40:4745-61. [Crossref] [PubMed]
- Catarci M, Benedetti M, Maurizi A, et al. ERAS pathway in colorectal surgery: structured implementation program and high adherence for improved outcomes. Updates Surg 2021;73:123-37. [Crossref] [PubMed]
- Ficari F, Borghi F, Catarci M, et al. Enhanced recovery pathways in colorectal surgery: a consensus paper by the Associazione Chirurghi Ospedalieri Italiani (ACOI) and the PeriOperative Italian Society (POIS). G Chir 2019;40:1-40. [PubMed]
- Tesauro M, Guida AM, Siragusa L, et al. Preoperative Immunonutrition vs. Standard Dietary Advice in Normo-Nourished Patients Undergoing Fast-Track Laparoscopic Colorectal Surgery. J Clin Med 2021;10:413. [Crossref] [PubMed]
- Xu D, Li J, Song Y, et al. Laparoscopic surgery contributes more to nutritional and immunologic recovery than fast-track care in colorectal cancer. World J Surg Oncol 2015;13:18. [Crossref] [PubMed]
- Sileri P, Mele A, Stolfi VM, et al. Medical and surgical treatment of chronic anal fissure: a prospective study. J Gastrointest Surg 2007;11:1541-8. [Crossref] [PubMed]
- Biancone L, Onali S, Calabrese E, et al. Non-invasive techniques for assessing postoperative recurrence in Crohn’s disease. Dig Liver Dis 2008;40:S265-70. [Crossref] [PubMed]
- Gentileschi P, Camperchioli I, Benavoli D, et al. Laparoscopic single-port sleeve gastrectomy for morbid obesity: preliminary series. Surg Obes Relat Dis 2010;6:665-9. [Crossref] [PubMed]
- Siragusa L, Pellino G, Sensi B, et al. Ambulatory laparoscopic colectomies: a systematic review. Colorectal Dis 2023;25:1102-15. [Crossref] [PubMed]
- Siekmann W, Eintrei C, Magnuson A, et al. Surgical and not analgesic technique affects postoperative inflammation following colorectal cancer surgery: a prospective, randomized study. Colorectal Dis 2017;19:O186-95. [Crossref] [PubMed]
- Pędziwiatr M, Pisarska M, Major P, et al. Laparoscopic colorectal cancer surgery combined with enhanced recovery after surgery protocol (ERAS) reduces the negative impact of sarcopenia on short-term outcomes. Eur J Surg Oncol 2016;42:779-87. [Crossref] [PubMed]
- Dodson S, Baracos VE, Jatoi A, et al. Muscle wasting in cancer cachexia: clinical implications, diagnosis, and emerging treatment strategies. Annu Rev Med 2011;62:265-79. [Crossref] [PubMed]
- Evans WJ. Skeletal muscle loss: cachexia, sarcopenia, and inactivity. Am J Clin Nutr 2010;91:1123S-7S. [Crossref] [PubMed]
- Lieffers JR, Bathe OF, Fassbender K, et al. Sarcopenia is associated with postoperative infection and delayed recovery from colorectal cancer resection surgery. Br J Cancer 2012;107:931-6. [Crossref] [PubMed]
- Zhang FM, Ma BW, Huang YY, et al. Laparoscopic colorectal cancer surgery reduces the adverse impacts of sarcopenia on postoperative outcomes: a propensity score-matched analysis. Surg Endosc 2020;34:4582-92. [Crossref] [PubMed]
Cite this article as: Guida AM, Franceschilli M, Leonetti G, Bellato V, Pirozzi BM, Fiorani C, Siragusa L, Sica GS. Pathophysiology of metabolic changes and malnutrition in colorectal cancer’s patients. AME Med J 2025;10:13.