Anesthesia for electrophysiology procedures
Introduction
The electrophysiology (EP) lab is a Non-Operating Room Anesthesia (NORA) site. The American Society of Anesthesiologists (ASA) closed claims database shows that 25% of claims occur in remote locations including cardiology catheterization and EP laboratories. Events include airway injury, permanent brain damage and death (1,2). The ASA has published guidelines for NORA to improve patient safety and outcomes which include recommendations for minimum equipment and monitoring in line with operating room-based anesthetics as well as specific mandates for radiation protection (2).
Patients undergoing EP procedures range from relatively healthy young patients having a supraventricular tachycardia (SVT) ablation to those with severe cardiac dysfunction necessitating a ventricular tachycardia (VT) ablation or implantable cardioverter defibrillator (ICD) implantation. In addition to worsening patient comorbidities, EP procedures can cause cardiac perforation and tamponade. An example of this is a lead extraction procedure; unlike a lead explant, a lead extraction involves removal of a lead that was implanted at least one year prior, or requires unique tools that are typically not required for lead implantation (3). Anesthesiologists with cardiac training and expertise in transesophageal echocardiography (TEE), cardiac surgeons, surgical scrub teams and perfusionists should be available for procedures in which emergent sternotomy and initiation of cardiopulmonary bypass (CPB) may be needed (1).
This review discusses the unique procedures common to the EP lab and the anesthetic management of patients with minimal to more severe cardiac dysfunction. We will review implanted electronic devices, ablation of various atrial and ventricular arrhythmias, cardioversion, lead extractions, left atrial appendage (LAA) exclusion devices, and emerging hybrid procedures. We feel this review is a most comprehensive summary of the literature including both frequently encountered electrophysiology cases as well as the newest emerging technologies and hybrid procedures.
Cardiac implantable electronic devices (CIEDs)
Pacemakers and ICD
CIEDs manage a broad range of cardiac electrical disturbances and can improve cardiac performance in patients with depressed ventricular function. Pacemakers treat tachycardic and bradycardic arrhythmias. Cardiac resynchronization therapy (CRT) devices are capable of biventricular pacing and improving left ventricular (LV) function. ICDs treat severe ventricular arrhythmias (4).
It took nearly a decade from the first pacemakers in the 1950s to allow patients mobility rather than being tethered to their devices via an extension cord (4). Since that time, devices have become smaller with lengthier battery life and recent advances have led to leadless devices and incorporation of wireless monitoring and programming technology. ICDs have also seen a transition from traditional transvenous access to subcutaneous lead placement with a similar role for wireless technology. Batteries may eventually become obsolete with development of an ability to convert cardiac motion to electrical energy (4).
CIED leads are most commonly placed transvenously, usually utilizing the left axillary or subclavian vein with a small 4–6 cm incision and subcutaneous pocket for pulse generator placement. Cephalic vein access has also been described. Central venous guide wires are placed under fluoroscopic and/or ultrasound guidance followed by a sheath through which leads are inserted (5). The leads connect to the myocardium via active fixation, where the helical lead tip is screwed directly into the myocardium, or passive fixation, where tines hold the lead in place by “grabbing” the trabeculated myocardium (6). Complications are similar to other transvenous procedures in this region and include vascular injury and pneumothorax in the setting of subclavian access. Lead placement may also cause cardiac perforation and pericardial tamponade.
CRT devices improve cardiac function in patients with reduced LV systolic function and interventricular conduction delay by synchronizing ventricular depolarization with a right ventricular (RV) lead and a LV pacing lead placed via the coronary sinus (4). CRT may also be obtained via His bundle or left bundle branch pacing. In addition to the above-mentioned complications, CRT devices are also associated with coronary sinus dissection.
ICDs are designed to treat VT with rapid ventricular pacing and defibrillation. The pulse generators are larger than pacemakers and require a capacitor to store energy prior to the electrical shock. The RV leads have a large-surface coiled electrode positioned in the RV apex (5). ICDs have pacing capabilities and antitachycardia pacing to treat bradycardia and ventricular arrhythmias, respectively. Devices with ICD and CRT therapies (CRT-D) are available to treat arrhythmias and improve LV function.
A review of observational studies using a procedural-based nurse (RN) administration model by Gerstein et al. did not find conclusive evidence to comment on the superiority of this practice over the inclusion of anesthesiology providers (7). They did conclude that there were overall more complications with older, sicker patients and longer procedures and did report that anesthesiology participation would be appropriate in these cases (7). A further retrospective review studied nearly 600 pacemaker and ICD implantations with propofol sedation administered by RNs who were trained with an education protocol. In this study, 10% of patients required positive pressure ventilation, vasopressor support, or an unanticipated ICU admission. In 38% of cases, minor events occurred including hypotension or the need of oral airway placement (8). Finally, a retrospective examination of the National Anesthesia Clinical Outcomes Registry from 2010 to 2021 showed that approximately 50% of ICD cases utilized general anesthesia instead of sedation (9).
While some centers rely on local anesthetics and nurse-administered sedations, in many academic medical centers, a team-based model with a cardiac anesthesiologist and a nurse anesthetist is employed. Local anesthetics with a long half-life (Table 1) are usually infiltrated into the subcutaneous pocket by the cardiologist, providing procedural and postoperative pain relief. The anesthesia team frequently utilizes propofol administered in small boluses or as a continuous infusion (50–125 mcg/kg/min) to achieve a level of deep sedation. Dexmedetomidine infusions can be used for lighter sedation. Benzodiazepines and opioids may be used when indicated.
Table 1
Drug | Concentrate (mg/mL) | Max dose by weight (mg/kg) | Total max dose (mg) | Max dose with epinephrine by weight (mg/kg) | Total max dose with epinephrine (mg) | Limit (mL) | Limit with epinephrine (mL) |
---|---|---|---|---|---|---|---|
Lidocaine 1% | 10 | 5 | 350 | 7 | 500 | 35 | 50 |
Mepivacaine 2% | 20 | 5 | 350 | 7 | 500 | 17.5 | 25 |
Bupivacaine 0.25% | 2.5 | 2 | 175 | 3 | 225 | 70 | 90 |
Ropivacaine 0.5% | 5 | 3 | 200 | 3.5 | 250 | 40 | 50 |
Leadless permanent pacemakers
Transvenous permanent pacemakers have complication rates of 10–20% incidence at 5 years (10). Leadless pacemakers have been developed to avoid many of these complications. In the United States, the Micra VR and the Micra AV (Medtronic, Minneapolis, MN, USA) and the Aveir VR (Abbott, Abbott Park, IL, USA) have been FDA approved for the treatment of bradyarrhythmias.
Both Micra devices consist of a small bipolar pacemaker capsule which adheres to RV myocardium via nitinol tines and can pace in VVI, VVIR, VOO and OVO modes with a battery life of up to 12 years (1). It has a small built-in accelerometer to provide heart rate responsiveness (11). The Micra AV adds VDD, VDI and ODO modes. Atrial sensing is provided by accelerometer detection of right atrial contractions (11). The MARVEL-2 study compared patients using VVI and VDD modes and found that the percentage of time spent in AV synchrony increased from an average of 26.8% in the former to almost 90% in the latter (12).
The Aveir VR is unique from the Micra by having a larger size, longer battery life (projected 10.2 vs. 4.7 years) (13) and a novel fixation helix with secondary anti-rotation tines (14). It can pace in VVI, VOO and OVO, and additionally has a magnet response to VVI (13). In a small retrospective analysis, the Aveir VR took longer to place and required twice the fluoroscopy time as the Micra VR (14). No difference was found in number of deployment/repositioning events or complications (14). The Aveir also has the ability to be used for dual chamber pacing if a second leadless pacemaker is placed at the base of the right atrial appendage with both implants communicating wirelessly (15). Recently, a prospective, multicenter-center trial was conducted with 300 patients and found that 97% of patients had a minimum of 70% atrioventricular (AV) synchrony at 3 months (14). The most common complications were AF and peri-procedure lead dislodgment. There were also two pericardial effusions and one retroperitoneal hematoma (14).
Implantation involves placement of a 27 French sheath in a femoral vein. Through this sheath, a delivery catheter is deployed and fluoroscopy is utilized to guide the leadless device through the right atria, across the tricuspid valve, and into position in the apical RV septum (16). An infusion of heparin is administered via a side port on the sheath to prevent thrombus; some suggest low dose systemic heparin while the sheath is in the femoral vein (16). At our facility, these cases are generally performed with anesthesia personnel providing sedation and electrophysiologist-administered local anesthetic infiltration at the access site. Beyond management of bradycardia prior to device placement, anesthesia considerations are similar to those of other transfemoral procedures.
Subcutaneous implantable cardioverter defibrillator (S-ICD)
The S-ICD was developed in order to avoid some of the complications of wired ICDs such as lead infection, migration, thrombosis, or damage to the tricuspid valve in patients who do not need bradycardia or antitachycardia pacing (10,17). In an examination of nearly 900 patients with an S-ICD, 90.1% of VT/ventricular fibrillation (VF) events responded to a single shock, while 98.2% of events were terminated with five shocks (18). While this was promising, the rate of inappropriate shock delivery in this same cohort was >13% (18).
The two approved models of the S-ICD are the Boston Scientific 1010 SQ-RX and the A209 EMBLEM. Both systems use a 45-cm subcutaneous electrode with proximal and distal sensing electrodes and an 8-cm defibrillation coil (18). These devices differ in their pulse generators and size, with the EMBLEM being slightly smaller and lighter (135 vs. 145 g) (18). The pulse generator is implanted in a subcutaneous pocket at the mid-axillary line at the 5th–6th intercostal space. Alternative placement options include the intermuscular plane between the serratus anterior and latissimus dorsi muscle (19). The electrode is tunneled medially to the xiphoid process and then in a superior direction where it lies anterior to the sternum (10).
An early experience with S-ICD placement began with all of the first four patients undergoing S-ICDs placements under conscious sedation by RNs; all of these patients reported severe pain during tunneling. In these four patients, there was also concern for airway compromise, LV dysfunction and hemodynamic instability with defibrillation threshold testing (DFT). As a result, the remaining 69 cases were performed successfully and safely under general anesthesia (20). Another study with anesthesia personnel performing deep sedation with propofol concluded that this technique provided patient comfort and maintained hemodynamic stability throughout tunneling and DFT (21). Regional anesthesia approaches require a dense blockade over a large area of chest wall and concerns over hemodynamic instability and anticoagulation limit the utility of paravertebral or thoracic epidural techniques (22). However, a combination of truncal plane nerve block techniques has been described (22). A panel of experts from high volume centers concluded that S-ICD placement requires deeper sedation than traditional ICD placement, but general anesthesia is not always necessary (22).
Transseptal puncture (TSP)
TSP is a crucial step in many ablative procedures. The technique involves obtaining left heart access for mapping and ablation by creating a hole in the interatrial septum which separates the right and left atria; this is functionally an iatrogenic atrial septal defect (ASD) (23,24). After obtaining venous access, wires are advanced into the right atrium. A sheath and specialized needles are then passed and the needle is used to puncture the atrial septum at the fossa ovalis, usually under fluoroscopic, TEE, or intracardiac echocardiography (ICE) guidance (23,24). A transseptal dilator and sheath are then placed and the needle is exchanged for guiding catheters which are advanced through the transseptal sheath to reach the left atrium (23,24). In most patients, the residual ASD will heal and seal off over time. As such, they are typically not empirically closed with occluder devices, though this may be indicated in patients with evidence of at risk for significant shunting (25). Complications of TSP are similar to other ablative procedures and include perforation of surrounding structures, including the atrial walls, the coronary sinus, and the aorta, with resultant pericardial tamponade (23,24). Given that there is left-sided arterial access after TSP, embolization of air or other debris is also possible (23,24).
Atrial fibrillation (AF) and atrial flutter (AFL) ablation
AF is the most common arrhythmia in the United States with a reported incidence of 0.4–1% worldwide (26). In contrast, AFL only affects approximately 0.1% of the world’s population (27). Risk factors for developing AF include cardiac disease, chronic pulmonary disease, smoking, hypertension, diabetes, and obesity (28).
Paroxysmal AF is defined as episodic events that spontaneously terminate within 7 days (29). Persistent AF lasts for at least 7 days, regardless of the use of pharmacologic treatment or cardioversion (29). Permanent AF is long-standing, persistent AF that has been sustained for greater than 1 year, in which cardioversion has failed or a decision has been made to not pursue rhythm control using electrical or ablative therapy (29).
The cardiomyocytes in the pulmonary veins play a central role in AF. These cells develop pacemaker-like activity resulting in spontaneous depolarization and/or micro-reentry circuits. Sustained AF can cause EP atrial remodeling, atrial dilatation and fibrosis, and progressive shortening of the refractory period leading to persistent AF (28,30). Recent evidence suggests than an increase in vagal tone can facilitate focal pulmonary vein electrical activity and affect both the atrial refractory period and the conduction velocity associated with AF (28). AFL is a macro-reentrant atrial tachyarrhythmia that usually arises from the right atrium. Typical AFL activates the right atrium counter-clockwise or clockwise about the tricuspid annulus with a critical region of slow conduction on the cavo-tricuspid isthmus (CTI). Atypical AFLs are observed in both right and left atria (31).
Catheter-based AF ablations require TSP and are usually done under general anesthesia to facilitate patient immobility and esophageal temperature monitoring. A randomized study found that general anesthesia had shorter fluoroscopy and procedure times than sedation (32). Furthermore, less patient movement and predictable diaphragm movement improved catheter stability and decreased the incidence of hemodynamic complications (32). There was also increased procedural success rate in patients under general anesthesia with a lower prevalence of pulmonary vein reconnection (32,33). Small boluses of short-acting opioids such as fentanyl may be used to manage pain during ablation of particularly painful areas including near the inferior vena cava (IVC) in typical AFL ablation and at the ostium of the left inferior pulmonary vein in AF ablation.
Typical AFL ablation is a right heart procedure which involves a linear ablation line along the CTI. Ablations for AFL are usually done under sedation (33). AFL ablation can be done anatomically without the need to induce arrhythmia during the procedure, which results in less risk of hemodynamic instability (33). Atypical AFL can be left-sided, requiring TSP and general anesthesia similar to AF ablation (33).
Cryoablation is an effective modality for pulmonary vein isolation (PVI). A “cryoballoon” is deployed into each pulmonary vein and a cryo refrigerant is used to inflate the inner balloon, causing a cryo thermal cellular injury, thus disrupting the aberrant AF pathway (34). Complications associated with cryoablation closely resemble those of traditional RFA, including tamponade and perforation, but the rate of phrenic nerve injury is significantly higher (1.7% vs. 0%) (34,35).
Pulsed-field ablation (PFA) is a newer ablation technique that creates cell membrane pores via irreversible electroporation, which specifically targets myocardial cells and causes cell death while sparing other types of cells, lowering the risk of phrenic nerve and esophageal injury. This technology also does not require fluid administration to irrigate and cool the ablation electrode, reducing the risk of volume overload and heart failure exacerbation in susceptible patients (36-38). Currently, there are no PFA systems that are FDA approved for the United States. Although multiple animal studies have shown PFA to be safer than thermal ablation procedures through reduced risk to surrounding structures, coronary vasospasm has been described during ablation of the mitral isthmus (39). General anesthesia is the preferred choice for PFA ablation, though there is emerging data suggesting that deep sedation with dexmedetomidine may be a viable alternative (40,41).
Monitoring and intraoperative management
Besides standard ASA monitors, radial artery catheterization is often used for blood pressure monitoring; however, noninvasive finger cuff arterial blood pressure monitors have gained popularity for ease of use, time savings, reliability, and adequate correlation with invasive monitoring (42). Esophageal temperature monitoring is routinely employed in patients undergoing RFA or cryoenergy ablations to prevent thermal injury to the esophagus during posterior ablation.
TEE has been used to rule out LAA thrombus especially if the patient has not been on anticoagulants. A large observational study in patients on uninterrupted direct oral anticoagulants for at least 4 weeks prior to ablation revealed a low incidence of thromboembolic events (43). The 2017 expert consensus statement on catheter and surgical ablation of AF gives a class IIa recommendation for performing a TEE in patients who are in AF on presentation, even if they have been receiving therapeutic anticoagulation for 3 weeks or longer (44). However, the 2020 European Society of Cardiology guidelines recommend therapeutic oral anticoagulation for at least 3 weeks prior to ablation (class I) or use of TEE to exclude LAA thrombus before ablation (45). In our institution, TEE evaluation of LAA for thrombus is not routinely employed in patients who have been on uninterrupted anticoagulation for more than three weeks and are also in normal sinus rhythm on the day of procedure.
Conventional ventilation under general anesthesia is using standard positive pressure ventilation with tidal volumes of 5–8 mL/kg ideal body weight and minimal positive end-expiratory pressure (PEEP). Neuromuscular blocking agents may be used to maintain patient immobility and consistent diaphragm movement; however, it may need be reversed during diaphragm pacing in order to monitor for phrenic nerve injury. High frequency jet ventilation (HFJV) may be used during ablation procedures in some centers to reduce respiratory excursion and motion to maintain ablation catheter stability during the procedure (46). First reported by Goode et al. in patients undergoing PVI for AF ablation in the early 2000s, small tidal volumes are introduced at relatively high frequencies of 100–130 “jets” or breaths/min through a stiff cannula or catheter (47). Because of the HFJV, the gases must be humidified to prevent mucosal drying/trauma. End-tidal CO2 (EtCO2) can be measured transcutaneously. It is important to establish arteriolar to EtCO2 gradient in order to use the EtCO2 to monitor ventilation. The most common complications of HFJV are hypercarbia and eventually hypoxemia. This can lead to systemic acidosis, right heart strain, and hypotension. As such, HFJV is contraindicated in patients with chronic obstructive pulmonary disease (COPD), pulmonary hypertension and increase intracranial pressure which all may be worsened with hypercarbia and hypoxemia. Barotrauma from the driving pressure can result in pneumothorax, pneumomediastinum, pneumopericardium, and subcutaneous emphysema.
A multidisciplinary approach to EP procedures is critical to provide comprehensive, well-coordinated care which is tailored to individual needs. Communication between anesthesiologists, electrophysiologists, and technicians is vital in coordinating appropriate timing of anesthesia induction and the mapping and ablation phases. Further anesthesiologists are essential in managing medications, anticoagulation, reliable temperature monitoring as well as critical events. Cardiac anesthesiologists are additionally valuable in their ability to provide procedural guidance and emergent imaging via TEE.
Complications
Open irrigation RFA is associated with a volume load of 17–30 mL/min, which can exacerbate heart failure (48). In patients undergoing PVI, the ablative catheters are in close proximity to the esophagus during ablation of the posterior wall of the left atrium (49). A randomized trial of capsule endoscopy in patients undergoing AF ablation found a higher incidence of esophageal injury in patients who received general anesthesia when compared to those who received sedation (50). This may have been due to reduced esophageal motility and deglutition under general anesthesia, which could result in ablation at the same esophageal location during the procedure (50).
A randomized study showed that active esophageal cooling may reduce the incidence of thermal injury to the esophagus during ablation procedures (51). Use of multisensor temperature probes have shown better sensitivity to temperature changes compared to single-sensor probes (52). Other measures to reduce esophageal injury include reducing the amount of RF power delivered, shortening the RF application time, decreasing contact force, and esophageal deviation. Mechanical esophageal deviation has been shown to reduce the rate of posterior atrial wall pulmonary vein reconnections compared to temperature monitoring alone (53).
The right phrenic nerve can be injured during ablation along the posterolateral wall of the right atrium and the right superior pulmonary vein (54). The left phrenic nerve can be injured during ablation near the LAA or over the epicardium of the LV (54). Injury to these nerves can be avoided with mapping using high output pacing from the ablation catheter to ensure a safe distance from the nerve. It is therefore important to avoid or reverse neuromuscular blockers in patients during pacing of the diaphragm.
The incidence of vascular complications following AF ablations ranges from 1–13% (55). Mechanical trauma from catheter manipulation during PVI is a possible mechanism for pulmonary hemorrhage (56). Cardiac tamponade has a reported incidence of 1% during PVI procedures (57). Thromboembolism can occur with placement of the sheaths and catheters in the arterial circulation. Heparin is usually administered before TSP and an activated clotting time of >300 s is maintained (1). However, there is a reported incidence of stroke in up to 5% of patients undergoing AF ablation despite perioperative anticoagulation (1,15,58,59), though modern data would support a 1% or less risk. After successful ablation and reversal of anticoagulation, patients must lie flat for 2–4 h to prevent access site bleeding. Groin sites should be monitored for retroperitoneal hematoma and can manifest as delayed refractory hypotension in the PACU.
Direct current cardioversion (DCCV)
Perhaps the most fundamental of EP procedures, DCCV of AF and AFL is a brief procedure for atrial and ventricular tachyarrhythmias. Patients are required to be on anticoagulants prior to elective cardioversion. If doses of these drugs have been missed, a TEE exam combined with cardioversion is required. If the patient has not yet started on anticoagulants, cardioversion can still be performed within 48 h of the known onset of the arrhythmia without a TEE exam.
Deep sedation is required for DCCV. In patients with devices that can pace the atrium, antitachycardia pacing at heart rates 10–20% faster than the intrinsic atrial rate can sometimes convert AFL. Typically, a bolus of a short-acting anesthetics including propofol, methohexital, or etomidate are used to facilitate a brief unconsciousness (60,61). A study comparing methohexital (0.5 mg/kg) and propofol (0.8 mg/kg) found that methohexital provided significantly faster recovery times, more stable hemodynamics, and less hypoxemia than propofol (62). Occasionally, fentanyl and midazolam may be used, particularly in an RN-based sedation model, but these medications are prone to a comparatively prolonged duration of effect (60). The mode of sedation is variable depending on patient characteristics. Airway obstruction is not unusual and a light jaw lift helps maintain a patent airway and prevent hypoxemia. An intriguing emerging medication that may have utility in DCCV is remimazolam, which combines the benzodiazepine effect of midazolam with the rapid, organ-independent metabolism of remifentanil to facilitate both rapid onset and emergence (63,64).
Of primary concern in these brief anesthetics is hemodynamic stability, with a sizeable fraction of patients having acute or acute on chronic cardiac dysfunction related to their arrhythmia (61). Patients with very rapid ventricular responses may have marginal blood pressures and require a small dose of vasopressor with the anesthetic bolus. Hypotension may also be common after cardioversion if the patient has sinus bradycardia. Occasionally, a prolonged sinus arrest may require transcutaneous pacing until sinus rhythm returns. Despite returning to sinus rhythm, atrial contractility may not be effective for hours to days after cardioversion. In patients with LV dysfunction in AF, functional recovery of ejection fraction occurs up to 4 weeks after cardioversion (65).
Supraventricular arrhythmia ablation
SVTs are a frequently encountered arrhythmia in the EP lab, typically producing heart rates of 150–250 beats per minute (66). Atrioventricular nodal reentrant tachycardia (AVNRT) is the second most common indication for ablation after AF, followed by AFL, AV reentrant tachycardia (AVRT) and ectopic atrial tachycardia.
AVNRT is an arrhythmia that arises from a re-entry circuit within the AV junction which allows multiple conduction pathways, though the exact anatomic correlate to the circuit(s) remains unclear (67). Compared to antiarrhythmic medications, catheter ablation of AVNRT as a first-line treatment has significant benefit in reducing hospital admission and improving quality of life and conveys a success rate of approximately 95% with a single digit rate of recurrence and minimal risk of inadvertent AV nodal block (67,68).
AVRTs, including those with Wolff-Parkinson White (WPW) syndrome have a reentrant circuit comprised of two limbs, including the AV nodal-His Purkinje System and a second accessory pathway or “bypass tract” (67). This second tract is comprised of myocardial cells which directly link atrial and ventricular myocardium, bypassing the typical conduction pathway and exhibiting rapid conduction in most cases (69,70). The majority of these bypass tracts are located along the mitral valve and left free wall, with others in the septum or right free wall (71-73). Patients with symptomatic and recurrent AVRT are likely to benefit from catheter ablation with a similar high success rate and low complication rate to AVNRT ablation (67).
As SVTs are prone to influence and obscuration by sedating medications, most SVT ablation procedures are performed under light sedation using intravenous infusions and monitored anesthesia care (MAC) (66). This should be tailored to the specific needs of the patient and the procedure. Most paroxysmal SVT ablations are performed with RFA, although focal cryoablation is also used when ablation is performed in pediatric patients, and/or the ablation target is close to critical structures such as the phrenic nerve or compact AV node. This is because the cyro-lesion can often be terminated when evidence of collateral damage is noted but before permanent injury occurs, albeit with the trade-off of a higher recurrence rate compared to RFA (74). Electroanatomic mapping is commonly used to reduce fluoroscopy and facilitate arrhythmia diagnosis and ablation (75).
Ventricular arrhythmia ablation
The two most common EP procedures for ventricular arrhythmias are ablation of premature ventricular complexes (PVCs) and VT. The anesthetic management of these procedures requires selecting an anesthetic that provides adequate levels sedation while allowing for effective electroanatomic mapping of the focus (76). General anesthesia with intravenous and volatile agents (76,77), deep versus minimal sedation (78), and specific sedation protocols using both sufentanil (79), and remifentanil (80) in combination with midazolam have been studied. Recently, a study conducted during the coronavirus disease 2019 (COVID-19) pandemic (where airway manipulation was avoided) found that PVC ablations under local anesthetic only were associated with a higher acute ablation success rate than those performed under general anesthesia (81). This supports the argument that any type of intravenous or inhaled anesthetic might reduce stress release of catecholamines and make mapping less successful. Moreover, isoproterenol might be required intravenously to elicit arrhythmias that might be adrenergically-mediated. Another issue is the use of neuromuscular blockade during ventricular ablation procedures. Phrenic nerve function is typically monitored intraoperatively during procedures near the nerve, like epicardial ablations, as noted above (76,82).
A joint consensus statement on catheter ablation of ventricular arrhythmias published by the Heart Rhythm Association and the European Heart Rhythm Association in 2019 recommends that the depth and type of anesthetic should be tailored specifically to the patient and procedure. For procedures targeting idiopathic ventricular arrhythmias, especially catecholamine-mediated, avoidance of both general anesthesia and deep sedation is recommended. Conversely, deep sedation or general anesthesia are reasonable options for longer procedures targeting scar-mediated arrhythmias or for procedures requiring more stimulating or painful access, such as subxiphoid epicardial approaches (82). Moreover, the anesthesia provider must be prepared to alter the type and depth of anesthetic intraoperatively based on whether mapping or ablation is being performed (76,82). Additionally, the patients should be aware that reducing the depth of anesthesia might be necessary to localize the area of the inciting focus.
Another consideration pertinent to ventricular ablation procedures involves anticipating periods of hemodynamic instability during VT, which would demand pharmacologic or mechanical hemodynamic support. Vasoconstrictive agents such as norepinephrine and phenylephrine are often used to maintain adequate perfusion pressures. Agents such as epinephrine and dobutamine are usually avoided due to their chronotropic actions; however, isoproterenol may be needed to identify a ventricular focus.
When significant or prolonged hemodynamic compromise is anticipated during a ventricular ablation procedure, particularly in patients with depressed ventricular function, temporary mechanical support devices can be placed perioperatively. Commonly used devices include percutaneous left ventricular assist devices (pLVADs) and extracorporeal membrane oxygenation (ECMO). Intra-aortic balloon pumps appear to offer fewer advantages in the setting of prolonged VT and generally are not preferred as support devices during ablation procedures (82,83).
Of note, advances in technology have resulted in the development of multiple new VT mapping techniques, to include decrement evoked potential (DEEP), isochronal late activation mapping (ILAM), and ripple mapping (RM). These newer techniques have the potential advantage of identifying targets for ablation without inducing VT, thereby reducing both procedural length and the risk of hemodynamic instability during VT ablations (84). As mapping and ablation technologies continue to evolve, anesthetic care for patients undergoing these procedures will require careful consideration of both patient and procedural complexities.
Laser/mechanical lead extraction
The Heart Rhythm Society defines a lead explant as when the leads are less than one year old and no special tools are involved in removal. Leads implanted for a longer period of time may have developed fibrotic adhesions. A lead extraction is defined as removal of a lead that has either been implanted for at least 1 year, or removal that requires using specialized tools (3). The most common scenarios for transvenous lead extraction are CIED infection or lead dysfunction (85). Other possible indications include addition of leads in the setting of an occluded venous system, superior vena cava (SVC) stenosis, significant arrhythmias, severe tricuspid regurgitation, chronic pain due to the leads, lead thrombosis, or lead recall.
Preoperatively, a history and physical is paramount and can be helpful in assessing risk factors for periprocedural complications (Table 2). A patient with complete heart block may require temporary pacing once the pacing lead is removed (86). Similarly, knowing of a history of multiple defibrillations from the CIED is helpful when assessing risk for significant arrhythmias perioperatively. The most recent echocardiogram should be reviewed to evaluate cardiac function, valvular competence, and the presence of any vegetations and/or thrombus. A chest X-ray can also be helpful to observe the number and location of the leads (86).
Table 2
Patient factors | CIED/institutional factors |
---|---|
Female sex | Pacemaker lead >10 years old |
Increased age | ICD lead >10 years old |
Severely reduced LVEF | ICD lead shaped flat, small diameter, or dual coil |
Kidney dysfunction | >2 leads extracted |
History of stroke | CIED lead infection |
BMI <25 kg/m2 | Low volume center/low proceduralist experience |
Anemia | |
Diabetes mellitus |
CIED, cardiovascular implantable electronic device; ICD, implantable cardioverter-defibrillator; LVEF, left ventricular function; BMI, body mass index.
Prior to starting the procedure, a multidisciplinary team discussion is advantageous to ensure the presence of required equipment and personnel and a contingency plan. The availability of a cardiothoracic surgical backup team, a perfusionist, a CPB circuit, pacing and/or defibrillation equipment, a SVC balloon, and crossmatched blood (87) should be discussed. At our institution, a cardiac anesthesiologist is involved with these cases, a specific cardiac surgeon is designated as backup, surgical scrub nurses are present in the room, and a CPB circuit is immediately available outside the room.
The European Heart Rhythm Association does not recommend a specific type of anesthesia, although they do state that assistance from anesthesia must be immediately available if the procedure is not performed under general anesthesia (88). Similarly, the Heart Rhythm Society in collaboration with the ASA did not recommend a specific type of anesthesia in their expert consensus document. However, they do state that most centers use general anesthesia, which has the advantages of patient comfort, a secure airway, and the ability to use TEE for monitoring (3). In a large European registry, general anesthesia was used in 38.7% of cases, sedation was used in 30.7%, and the remaining 30.6% of cases were performed under local anesthesia alone (89). In a recent United States registry analysis, it was noted that use of MAC for ICD lead extractions has trended down from a rate of 31.25% in 2010 to 7.62% in 2021 (9).
It is our institutional practice to perform lead extractions under general anesthesia. In additional to standard ASA monitors, an arterial line is also placed. It may be preferable to place the arterial line on the opposite side of the CIED to prevent erroneous readings from manual pressure placed on the subclavian artery during the procedure (85). Large bore intravenous access allows for adequate resuscitation if bleeding occurs. The location of the intravenous line may also be important depending on if a venogram is required or if there is potential to use an SVC balloon. The SVC balloon is an endovascular treatment option that is advanced from the femoral vein to limit bleeding by rapid occlusion of the SVC in the event of an SVC tear (90). If an SVC balloon is being considered, a dedicated femoral venous line should be placed since upper extremity intravenous access may be of limited use during SVC occlusion. TEE allows for continuous cardiac monitoring and can be particularly useful for evaluation of thrombus, vegetations, tricuspid regurgitation before and after lead extraction, and identification of complications such as a new pericardial effusion (85).
To provide optimal care, the anesthesiologist should have a general understanding of the lead extraction procedure. Briefly, the pocket is opened, and the leads are isolated after dissection of scar tissue. The lead(s) is then disconnected from the connection block of the generator. Thereafter, some leads can be removed with simple traction, particularly if the leads have only been in place for a short period of time (91). If this strategy is not effective, the traction can be repeated with use of a locking stylet. If still unsuccessful, there are several sheaths that can be used. A simple telescoping sheath is unpowered and allows for manual disruption of adhesions. Alternatively, powered options include a laser sheath as well as a mechanical rotating sheath to cut though fibrosis. Notably, interventionists are not limited to one option alone and may use a combination of techniques (Figure 1) (91,92).
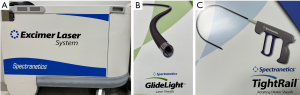
Major complications are uncommon and are estimated to occur in roughly 2% of cases (93). These may include cardiac perforation, vascular tears (such as SVC), hemorrhage, cardiac tamponade, significant tricuspid valve injury, and death (94). However, complications may vary with the level of experience. Maus et al. reported a major complication rate of 12.12% during their first year; thereafter, however, it decreased to 1.23% over the next few years (95). Since experience is important, the Heart Rhythm Society recommends that physicians extract a minimum of 40 leads during training and perform at least 20 extractions per year (3). While uncommon, major complications can result in death quickly, which highlights the importance of cardiac surgical backup being immediately available. Complications are summarized in Table 3.
Table 3
Hemorrhage |
Vascular tear (such as superior vena cava) |
Cardiac perforation/tamponade |
Tricuspid valve injury |
Death |
LAA occlusion/exclusion devices
Patients with AF are at increased risk of thrombus formation and a 5-fold increased risk of stroke. Strokes attributed to AF are more severe and lead to greater disability than stroke from another etiology (96). Several anticoagulants have been identified as effective in preventing stroke in patients with AF (97). However, the risk of bleeding complications, a narrow therapeutic window and potential for non-compliance has spurred an interest in LAA occlusion (98). The PROTECT-AF trial has shown LAA occlusion device is non-inferior to Coumadin for stroke prevention (99). The PREVAIL trial showed a 96% success rate in device placement and a 4% complication rate in the first week (100). Percutaneous LAA closure devices are being done in greater number and with improving complication rates (101).
In addition to the typical preoperative assessment, there are several items to consider for patients prior to LAA occlusion. Perioperative anticoagulation/antiplatelet management should be discussed with implanting physician (96). Renal function should be assessed because intravenous contrast is used during the procedure. Concerns regarding renal function should be discussed with the proceduralist to minimize contrast exposure and contrast-associated renal injury (96). CT imaging is done to assess the dimensions of the LAA prior to the procedure. If thrombus is noted in the LAA, serial computed tomography or TEE is done to confirm resolution of clot before procedure with LAA closure.
General endotracheal anesthesia is commonly used to facilitate the use of TEE throughout the procedure. General anesthesia provides akinesia, which helps reduce the risk of LAA perforation during device placement (98). MAC cases with deep sedation cases have been reported. A case series of 11 patients who underwent LAAO with TEE guidance under MAC reported a 100% success rate for device placement. However, there was no comparison to a GETA group (102). A study comparing GETA versus MAC had an implant success rate of 93.9% and 96.9%, respectively. The major periprocedural complication rate for GETA was 3.5% and 2.5% for MAC. These differences were not statistically significant in the study of over 500 patients (103). Use of ICE can mitigate the need for intraprocedural TEE and therefore be more amenable to MAC anesthetic (104). Patient factors to consider if deciding between general anesthesia and MAC include the ability to lie flat, obstructive sleep apnea risk, aspiration risk, difficulty of cannulation, cardiologist experience, and institutional complication rate (98).
Anesthesiologists need to be cognizant of the potential complications of the procedure when forming the anesthetic plan. Major risks include pericardial effusion, vascular injury leading to hemorrhage, stroke, and device embolization. In the PREVAIL trial, 3,800 patients received a LAA occlusion device with complication rates of 1% for tamponade, 0.4% for bleeding requiring transfusion, 0.25% for device embolization, 0.08% for stroke, and a 0.08% mortality rate (100).
Standard ASA monitoring is expected for these procedures. Invasive arterial pressure monitoring is commonly used due to the risk of arrhythmias and pericardial effusion. Large bore peripheral access or central venous access is advised. A left atrial pressure >10 mmHg is preferred to avoid under sizing the LAA occlusion device (97), which can be achieved with intravascular volume. Similarly, the availability of blood products for the patient should be established prior to the procedure (96). A type and screen will identify patients with pre-existing antibodies and allow for appropriate planning to have adequate blood available.
Measurements of the LAA usually commence after anesthesia and placement of the TEE probe. At least 4 different views (0°, 45°, 90°, 135°), are used to evaluate the LAA for ostium size, depth and shape. Ostial measurement is done from the superior portion of the mitral annulus to the limbus of the left upper pulmonary vein or to the ligament of Marshall. The largest ostial diameter measurements are commonly at 0° and 135° (96). The diameter of the device is also the same as the depth. LAA depth needs to be greater than the ostial diameter to ensure maximum device diameter is distal to the ostium.
Percutaneous venous access is obtained, commonly in a femoral vein. A catheter is advanced from the femoral vein to the right atrium near the interatrial septum. Under fluoroscopic and/or TEE guidance, the septum is punctured to access the left atrium. Puncture at the posterior portion of the septum is optimal (97). Identification of anterior and posterior septum can be identified via a midesophageal view at 45° omniplane where the aortic valve is visible. In contrast, the relationship of inferior and superior septum is best seen in the midesophageal bicaval view.
If heparin has not already been administered, it should be given after transeptal puncture. The goal activated clotting time is institution dependent, but within the range of 200–300 is an acceptable value (96,97).
A stiff wire is then advanced into the left upper pulmonary vein followed by a guiding catheter placed over the wire. A pigtail catheter is advanced through the guiding catheter and into the LAA. Once position is confirmed in the LAA, the guiding catheter is advanced to the os of the LAA, and the pigtail catheter is removed. The delivery catheter is then inserted and advanced into the LAA. The occlusion device is then unsheathed but remains attached to the delivery system (105). Angiography and TEE are used to assess adequacy of placement. The maximum diameter of the device should be at or distal to the opening of the LAA (96,105). Adequate compression of the device within the LAA of 8–20% compression is recommended to avoid device embolization. Color Doppler interrogation is used to assess gaps between the LAA and the occlusive device. Any jet <5 mm is considered successful placement (96,97,105). Position, anchor, size and seal (PASS criteria) are checked prior to release (97). After deployment, the system is removed, protamine is administered, and hemostasis of venous access site is obtained. Transthoracic echocardiography can evaluate for effusion prior to discharge from the hospital. This exam can also be used to assess the LAA occlusion device via the subxiphoid view (96).
Hybrid procedures with cardiac surgeons
Convergent procedure
While the mainstay of interventional AF treatment remains endocardial catheter ablation due to its minimal invasiveness and overall reassuring risk profile, the lack of transmural ablation may limit its success, particularly in patients with recurrent AF (106,107). Surgical ablation at the time of other cardiac surgery has increased in frequency since its advent in the 1980s, but only 3% of patients with AF suffer from other conditions that merit surgical intervention themselves (107-109). The success of catheter ablation ranges from 20–50% for single ablations of persistent AF, the success rates of thoracoscopic or open surgical approaches are higher, and of lower risk to surrounding structures due to improved visualization and access to the posterior wall (106,107,110).
The hybrid convergent ablation (HCA) approach has been developed to combine the individual strengths of minimally-invasive endocardial and surgical epicardial ablation. The HCA procedure is a multidisciplinary endeavor where surgeons perform a variant of Cox-Maze epicardial ablation of the posterior left atrial wall via subxiphoid or transdiaphragmatic approaches followed by concomitant endocardial ablation and PVI via RFA catheters by electrophysiologists (110,111). This may be performed sequentially in a single procedure using a hybrid operating room setup or in a staged fashion (111).
In a randomized control trial, 74% subjects in HCA achieved at least 90% AF burden reduction. This was only achieved in 55% of subjects with endocardial catheter ablation alone (106). HCA has demonstrated improved duration of freedom from AF (FFAF), with 73% of HCA patients FFAF compared to 49% of endocardial ablation patients with HCA favored by an odds ratio of 2.78 in one meta-analysis (107). HCA demonstrates an approximately 18% absolute and 35% relative improvement over endocardial ablation alone (106). One cohort experienced 78% freedom from symptomatic AF at 1-year follow-up (110).
Anesthetic management for the convergent procedure combines the priorities of both the epicardial and endocardial portions. Logistical preparation includes coordination of anesthesia, electrophysiology, and surgical teams. Due to the potential for catastrophic bleeding during the procedure, it is imperative to establish large bore venous access pre-incision, either peripherally or centrally, and to have blood products as well as CPB and perfusion support immediately available. Peripheral arterial monitoring is also used in addition to standard monitors.
Due to the requirement for either laparoscopic or thoracoscopic access and patient immobility during ablation, general endotracheal anesthesiology is standard. Esophageal temperature monitoring is performed as noted above. In the case of concomitant LAA ligation, which may accompany the convergent procedure in some high-risk patients, lung isolation with either a double lumen endotracheal tube or bronchial blocker is indicated (109). Long-acting paralytics should be avoided during the intrathoracic portion so that phrenic nerve stimulation can be appreciated.
Inappropriate sinus tachycardia (IST) ablations
IST is defined as unexplained persistent or paroxysmal sinus tachycardia >100 beats per minute with an average 24-h heart rate >90 beats per minute. Palpitations are the most commonly reported symptom but other associated symptoms include fatigue, presyncope or syncope, shortness of breath, and chest pain (112-114). It is predominantly a disease of younger women between age 15 and 45 years, with an odds of 4:1 toward women versus men, though some elderly patients have been diagnosed in their 6th or 7th decade of life (113). It is thought to potentially be underrecognized in the general population and to be on the continuum of dysautonomia with postural tachycardia syndrome (POTS) and vasovagal syncope (112,113). It has been proposed that there may be several subsets of patients with IST depending on their degree of baseline tachycardia and variable response to stimuli (113). Rarely, these patients develop tachycardia-induced cardiomyopathy, but most cases are deemed benign apart from the potential impact on quality of life, which can be profound (112,114).
The pathogenesis of IST is poorly understood and is thought to be multifactorial with several proposed mechanisms (Table 4) (112,113,115,116). As a diagnosis of exclusion, potential orthostatic contribution as well as superimposed supraventricular or reentrant tachycardias and structural heart disease must be ruled out (112). As many patients with IST have concurrent psychiatric diagnoses, treatment of IST hinges primarily on psychiatric treatment, lifestyle modification, and trigger avoidance. Most patients unresponsive to these nonpharmacological interventions will trial calcium-channel or beta blockers, but these medications are usually incompletely effective and poorly tolerated (112,113). Ivabradine, a funny current (If) inhibitor, is emerging as a pharmacologic option, but is contraindicated in pregnancy and breastfeeding, which limits its utility in a swath of this population (112,113,117).
Table 4
Intrinsic sinus node dysfunction (increased sinus node automaticity) |
Lack of or impaired vagal parasympathetic inhibition of sinus node |
Beta receptor autoantibody or hypersensitivity (oversensitive sympathetic stimulation) |
Muscarinic 2 receptor autoantibody or hyposensitivity (impaired parasympathetic inhibition) |
Impaired baroreflex |
Central autonomic hyperactivity |
Aberrant neurohormonal modulation (histamine, serotonin, substance P, vasoactive intestinal peptide, etc.) |
Endocardial sinus node mapping and modification (SNM) via RFA has been deployed in this population for years but remains variably successful (112,113). As such, it is only reserved for patients which have failed all conservative measures with debilitating symptoms. Randomized control trial data to guide patient selection for procedures is lacking. Of note, the goal is not complete sinus nodal ablation, which would require permanent pacemaker placement, though this does occur inadvertently in up to 30% of patients (113,118).
The target of ablation is destruction of areas of early activation in sinus tachycardia and interruption of potential accessory inputs to the sinus node (112). Ablation is performed starting at the location of earliest activation in sinus rhythm. 3D mapping is commonly employed. RFA lesions are then carried cranially and/or caudally along the crista terminalis until a heart rate reduction has been achieved. The phrenic nerve location is mapped and avoided (119,120). The goal is to ablate the more cranial portions of the sinus node complex, allowing locations more caudal along the crista terminalis set the pace at a lower rate. Success of endocardial ablation has improved over time with approximately 60–90% of patients reporting acute improvement after the procedure, but long-term success is variable (113,121). A large single center experience showed no long-term benefit to SNM in 65% of patients (118). This is likely because of the diffuse nature of the sinus node tissue and complex autonomic influences which cause the tachycardia focus to migrate from the site of initial ablation to areas lower in the atrium or towards the AV junction (122). To further improve long-term ablative success and minimize the risk of needing re-ablation and permanent pacemaker support, minimally invasive epicardial and hybrid endocardial/epicardial approaches akin to convergent procedures are being increasingly employed to target the sinus node and/or associated structures, including the autonomic inputs and the atriocaval junctions in sinus node-sparing procedures (112,113).
Anesthesia for these procedures is similar to most other ablative procedures, but has not been described systematically in the literature. Patients undergoing intrathoracic epicardial ablation should have general anesthesia, but, similar to the convergent procedure, long-acting paralytics should be avoided when there is potential for inadvertent phrenic nerve ablation.
Strengths and limitations
This review summarizes the clinical experience provided by an anesthesia service for a busy electrophysiology service in the United States. The literature on this topic is sparse and there are few randomized control trials. Most of the literature includes case reports or case series or review articles and science related to EP procedures and the clinical difficulties various EP procedures may cause for sedation/anesthesia services. The strengths include the clinical applicability of these services, but do not include regional or local biases related to individual variability on how EP procedures and anesthesia for these procedures are performed nor articles on the topic after the publication date for this journal.
Conclusions
EP are increasing in frequency and complexity. Given that most EP labs are NORA locations, the intricacies of anesthetizing patients for these procedures are marked. This is compounded by the increasingly ill population for which we care in the EP lab, with a broadening set of procedures including cardioversion, device implantation, EP mapping studies, and ablative procedures, as well as increasingly complex lead extractions and hybrid surgical procedures. Table 5 summarizes key considerations for anesthesiologists for each of these types of cases. Understanding of these procedures and their potential complications helps anesthesiologists to develop an appropriate plan for patients.
Table 5
Procedure type | Typical anesthetic | Key considerations | Potential complications |
---|---|---|---|
CIEDs—transvenous access | MAC with propofol infusion or dexmedetomidine; some institutions use local anesthetics only or nurse-administered IV sedation (fentanyl, midazolam) | Left axillary, subclavian, or cephalic access with left chest device pocket | Vascular injury |
ICD will require test shock | Pneumothorax | ||
Perforation/tamponade | |||
Local anesthetic toxicity (see Table 1) | |||
Leadless pacemaker | MAC with propofol sedation and local infiltration | Femoral access, large sheath | Vascular injury |
Perforation/tamponade | |||
Local anesthetic toxicity | |||
Subcutaneous ICD | GETA or very deep sedation | For patients who do not require pacing | Lead malposition |
Generator placed in mid-axillary line at 5th–6th intercostal space with electrode overlying sternum | Soft tissue injury/hematoma | ||
Tunneling is very stimulating | |||
AF/AFL ablation | GETA for left-sided ablation; potentially MAC for right-side only ablation (typical AFL) | TEE may be needed to rule out LAA thrombus preoperative | Arrhythmia |
Maintain preoperative anticoagulation for at least 3 weeks | Fluid overload | ||
TSP for left-sided ablation; heparinize to ACT >300 | Perforation/tamponade | ||
Esophageal temp monitoring is critical for posterior ablation | Esophageal ablation injury | ||
May need opioids at painful spots: LIPV in AF and near IVC in AFL | Embolization if TSP performed | ||
HFJV may be deployed to minimize respiratory excursion | Phrenic palsy with cryoablation | ||
Avoid neuromuscular blockade when ablating near phrenic nerve | Coronary vasospasm with PFA | ||
DCCV | Deep sedation with bolused propofol or methohexital versus RN-based sedation; remimazolam may become useful | Must be anticoagulated prior to procedure (unless within 48 h of onset) | Hypotension |
Maintain hemodynamic stability with bolused medication due to frequent concomitant cardiac dysfunction | Prolonged sinus arrest or symptomatic sinus bradycardia (may require transient TCP) | ||
SVT ablation | Local anesthetic; light to no sedation | Minimize sedation to avoid suppression of arrhythmia | Vascular injury |
Perforation/tamponade | |||
Local anesthetic toxicity | |||
PVC/VT ablation | Local anesthetic; light to no sedation to avoid suppressing arrhythmia | Minimize sedation to avoid suppression of arrhythmia | Prolonged unstable arrhythmia |
Isoproterenol may be used to induce arrhythmia | Vascular injury | ||
May require mechanical support (ECMO, percutaneous LVADs) in patients with depressed cardiac function | Perforation/tamponade | ||
Local anesthetic toxicity | |||
Laser/mechanical lead removal | GETA in most cases | Arterial line for close hemodynamic monitoring | Vascular injury (with access or SVC with removal) |
Large bore PIVs for resuscitation | Perforation/tamponade | ||
May need temporary backup pacing for pacer-dependent | Tricuspid valve injury | ||
Requires cardiac surgery and perfusion backup, crossmatched blood, SVC occlude balloon | Hemorrhage | ||
LAA occlusion/exclusion | Usually GETA for TEE guidance, but MAC with ICE has been used | Rule out LAA thrombus preprocedure with TEE or ICE | Vascular injury |
TEE for ostial and compression measurements | Perforation/tamponade | ||
Heparinization for TSP (ACT 200–300) | Device embolization | ||
Hybrid procedures (convergent) | GETA | Combined transvenous RFA ablation and surgical epicardial ablation | Vascular injury |
Arterial line for close hemodynamic monitoring | Perforation/tamponade | ||
Large bore PIVs for resuscitation | Arrhythmias | ||
Esophageal temp monitoring during RFA | Esophageal ablation injury | ||
Lung isolation for surgical exposure | |||
Avoid paralytics during intrathoracic portion for phrenic monitoring | |||
Inappropriate sinus tachycardia ablation | No systematic approach: local anesthetic; light to no sedation to avoid suppressing arrhythmia | Hybrid approaches are becoming common (similar setup to convergent procedure above) | Vascular injury |
Perforation/tamponade | |||
Accidental sinus node ablation | |||
Phrenic nerve injury |
EP, electrophysiologic; CIED, cardiac implantable electronic devices; MAC, monitored anesthesia care; GETA, general endotracheal anesthesia; ICD, implantable cardioverter defibrillator; AF, atrial fibrillation; AFL, atrial flutter; TSP, transseptal puncture; ACT, activated clotting time; LIPV, left inferior pulmonary vein; HFJV, high frequency jet ventilation; PFA, pulsed-field ablation; DCCV, direct current cardioversion; TCP, transcutaneous pacing; SVT, supraventricular tachycardia; PVC, premature ventricular contraction; VT, ventricular tachycardia; SVC, superior vena cava; PIV, peripheral intravenous access; LAA, left atrial appendage; ECMO, extracorporeal membrane oxygenation; LVAD, left ventricular assist device; TEE, transesophageal echocardiography; RN, procedural-based nurse; ICE, intracardiac echocardiography; RFA, radiofrequency ablation; IVC, inferior vena cava.
Acknowledgments
Funding: None.
Footnote
Peer Review File: Available at https://amj.amegroups.com/article/view/10.21037/amj-23-238/prf
Conflicts of Interest: All authors have completed the ICMJE uniform disclosure form (available at https://amj.amegroups.com/article/view/10.21037/amj-23-238/coif). R.L.R. serves as an unpaid editorial board member of AME Medical Journal from August 2023 to July 2025. R.J.F. received travel support from Abbott for MitraClip training. The other authors have no conflicts of interest to declare.
Ethical Statement: The authors are accountable for all aspects of the work in ensuring that questions related to the accuracy or integrity of any part of the work are appropriately investigated and resolved.
Open Access Statement: This is an Open Access article distributed in accordance with the Creative Commons Attribution-NonCommercial-NoDerivs 4.0 International License (CC BY-NC-ND 4.0), which permits the non-commercial replication and distribution of the article with the strict proviso that no changes or edits are made and the original work is properly cited (including links to both the formal publication through the relevant DOI and the license). See: https://creativecommons.org/licenses/by-nc-nd/4.0/.
References
- Fujii S, Zhou JR, Dhir A. Anesthesia for Cardiac Ablation. J Cardiothorac Vasc Anesth 2018;32:1892-910. [Crossref] [PubMed]
- Parameters ACoP. Statement on Nonoperating Room Anesthesia Services. American Society of Anesthesiologists, Washington, DC. 2023. Available online: https://www.asahq.org/standards-and-practice-parameters/statement-on-nonoperating-room-anesthesia-services. Accessed November 22, 2023.
- Kusumoto FM, Schoenfeld MH, Wilkoff BL, et al. 2017 HRS expert consensus statement on cardiovascular implantable electronic device lead management and extraction. Heart Rhythm 2017;14:e503-51. [Crossref] [PubMed]
- Albatat M, Bergsland J, Arevalo H, et al. Technological and Clinical Challenges in Lead Placement for Cardiac Rhythm Management Devices. Ann Biomed Eng 2020;48:26-46. [Crossref] [PubMed]
- Kotsakou M, Kioumis I, Lazaridis G, et al. Pacemaker insertion. Ann Transl Med 2015;3:42. [PubMed]
- Mulpuru SK, Madhavan M, McLeod CJ, et al. Cardiac Pacemakers: Function, Troubleshooting, and Management: Part 1 of a 2-Part Series. J Am Coll Cardiol 2017;69:189-210. [Crossref] [PubMed]
- Gerstein NS, Young A, Schulman PM, et al. Sedation in the Electrophysiology Laboratory: A Multidisciplinary Review. J Am Heart Assoc 2016;5:e003629. [Crossref] [PubMed]
- Sayfo S, Vakil KP, Alqaqa'a A, et al. A retrospective analysis of proceduralist-directed, nurse-administered propofol sedation for implantable cardioverter-defibrillator procedures. Heart Rhythm 2012;9:342-6. [Crossref] [PubMed]
- McGuire JA, Hayanga JWA, Thibault D, et al. Anesthetic Choice for Cardiovascular Implantable Electronic Device Placement and Lead Removal: A National Anesthesia Clinical Outcomes Registry Analysis. J Cardiothorac Vasc Anesth 2023;37:2461-9. [Crossref] [PubMed]
- Cronin B, Essandoh MK. Update on Cardiovascular Implantable Electronic Devices for Anesthesiologists. J Cardiothorac Vasc Anesth 2018;32:1871-84. [Crossref] [PubMed]
- Cody J, Graul T, Holliday S, et al. Nontransvenous Cardiovascular Implantable Electronic Device Technology-A Review for the Anesthesiologist. J Cardiothorac Vasc Anesth 2021;35:2784-91. [Crossref] [PubMed]
- Steinwender C, Khelae SK, Garweg C, et al. Atrioventricular Synchronous Pacing Using a Leadless Ventricular Pacemaker: Results From the MARVEL 2 Study. JACC Clin Electrophysiol 2020;6:94-106. [Crossref] [PubMed]
- Tang JE, Savona SJ, Essandoh MK. Aveir Leadless Pacemaker: Novel Technology With New Anesthetic Implications. J Cardiothorac Vasc Anesth 2022;36:4501-4. [Crossref] [PubMed]
- Tokavanich N, Machado C, Banga S, et al. Implant efficiency and clinical performance of Aveir™ VR and Micra™ VR leadless pacemaker: A multicenter comparative analysis of 67 patients. Pacing Clin Electrophysiol 2023;46:827-32. [Crossref] [PubMed]
- Knops RE, Reddy VY, Ip JE, et al. A Dual-Chamber Leadless Pacemaker. N Engl J Med 2023;388:2360-70. [Crossref] [PubMed]
- El-Chami MF, Roberts PR, Kypta A, et al. How to Implant a Leadless Pacemaker With a Tine-Based Fixation. J Cardiovasc Electrophysiol 2016;27:1495-501. [Crossref] [PubMed]
- Essandoh M, Daoud EG. Perioperative Considerations for Patients With Subcutaneous Implantable Cardioverter-Defibrillators Undergoing Noncardiac Surgery. J Cardiothorac Vasc Anesth 2016;30:756-61. [Crossref] [PubMed]
- Burke MC, Gold MR, Knight BP, et al. Safety and Efficacy of the Totally Subcutaneous Implantable Defibrillator: 2-Year Results From a Pooled Analysis of the IDE Study and EFFORTLESS Registry. J Am Coll Cardiol 2015;65:1605-15. [Crossref] [PubMed]
- Brouwer TF, Miller MA, Quast AB, et al. Implantation of the Subcutaneous Implantable Cardioverter-Defibrillator: An Evaluation of 4 Implantation Techniques. Circ Arrhythm Electrophysiol 2017;10:e004663. [Crossref] [PubMed]
- Essandoh MK, Portillo JG, Weiss R, et al. Anesthesia care for subcutaneous implantable cardioverter/defibrillator placement: a single-center experience. J Clin Anesth 2016;31:53-9. [Crossref] [PubMed]
- Essandoh MK, Otey AJ, Abdel-Rasoul M, et al. Monitored Anesthesia Care for Subcutaneous Cardioverter-Defibrillator Implantation: A Single-Center Experience. J Cardiothorac Vasc Anesth 2016;30:1228-33. [Crossref] [PubMed]
- Essandoh MK, Mark GE, Aasbo JD, et al. Anesthesia for subcutaneous implantable cardioverter-defibrillator implantation: Perspectives from the clinical experience of a U.S. panel of physicians. Pacing Clin Electrophysiol 2018;41:807-16. [Crossref] [PubMed]
- Almendarez M, Alvarez-Velasco R, Pascual I, et al. Transseptal puncture: Review of anatomy, techniques, complications and challenges, a critical view. Int J Cardiol 2022;351:32-8. [Crossref] [PubMed]
- Sharma SP, Nalamasu R, Gopinathannair R, et al. Transseptal Puncture: Devices, Techniques, and Considerations for Specific Interventions. Curr Cardiol Rep 2019;21:52. [Crossref] [PubMed]
- Drosos I, Rosa R, Seppelt PC, et al. Iatrogenic atrial septal defects after transseptal puncture for percutaneous left atrial appendage occlusion and their hemodynamic effects. J Geriatr Cardiol 2022;19:675-84. [PubMed]
- Chugh SS, Havmoeller R, Narayanan K, et al. Worldwide epidemiology of atrial fibrillation: a Global Burden of Disease 2010 Study. Circulation 2014;129:837-47. [Crossref] [PubMed]
- Granada J, Uribe W, Chyou PH, et al. Incidence and predictors of atrial flutter in the general population. J Am Coll Cardiol 2000;36:2242-6. [Crossref] [PubMed]
- Bhatt HV, Fischer GW. Atrial Fibrillation: Pathophysiology and Therapeutic Options. J Cardiothorac Vasc Anesth 2015;29:1333-40. [Crossref] [PubMed]
- January CT, Wann LS, Alpert JS, et al. 2014 AHA/ACC/HRS guideline for the management of patients with atrial fibrillation: executive summary: a report of the American College of Cardiology/American Heart Association Task Force on practice guidelines and the Heart Rhythm Society. Circulation 2014;130:2071-104. [Crossref] [PubMed]
- Allessie MA, Konings K, Kirchhof CJ, et al. Electrophysiologic mechanisms of perpetuation of atrial fibrillation. Am J Cardiol 1996;77:10A-23A. [Crossref] [PubMed]
- Yildiz M, Yilmaz Ak H, Oksen D, et al. Anesthetic Management In Electrophysiology Laboratory: A Multidisciplinary Review. J Atr Fibrillation 2018;10:1775. [PubMed]
- Di Biase L, Conti S, Mohanty P, et al. General anesthesia reduces the prevalence of pulmonary vein reconnection during repeat ablation when compared with conscious sedation: results from a randomized study. Heart Rhythm 2011;8:368-72. [Crossref] [PubMed]
- Alvarez CK, Zweibel S, Stangle A, et al. Anesthetic Considerations in the Electrophysiology Laboratory: A Comprehensive Review. J Cardiothorac Vasc Anesth 2023;37:96-111. [Crossref] [PubMed]
- Andrade JG, Wazni OM, Kuniss M, et al. Cryoballoon Ablation as Initial Treatment for Atrial Fibrillation: JACC State-of-the-Art Review. J Am Coll Cardiol 2021;78:914-30. [Crossref] [PubMed]
- Cardoso R, Mendirichaga R, Fernandes G, et al. Cryoballoon versus Radiofrequency Catheter Ablation in Atrial Fibrillation: A Meta-Analysis. J Cardiovasc Electrophysiol 2016;27:1151-9. [Crossref] [PubMed]
- Reddy VY, Anic A, Koruth J, et al. Pulsed Field Ablation in Patients With Persistent Atrial Fibrillation. J Am Coll Cardiol 2020;76:1068-80. [Crossref] [PubMed]
- Stewart MT, Haines DE, Verma A, et al. Intracardiac pulsed field ablation: Proof of feasibility in a chronic porcine model. Heart Rhythm 2019;16:754-64. [Crossref] [PubMed]
- Verma A, Boersma L, Haines DE, et al. First-in-Human Experience and Acute Procedural Outcomes Using a Novel Pulsed Field Ablation System: The PULSED AF Pilot Trial. Circ Arrhythm Electrophysiol 2022;15:e010168. [Crossref] [PubMed]
- Gunawardene MA, Schaeffer BN, Jularic M, et al. Coronary Spasm During Pulsed Field Ablation of the Mitral Isthmus Line. JACC Clin Electrophysiol 2021;7:1618-20. [Crossref] [PubMed]
- Grimaldi M, Quadrini F, Caporusso N, et al. Deep sedation protocol during atrial fibrillation ablation using a novel variable-loop biphasic pulsed field ablation catheter. Europace 2023;25:euad222. [Crossref] [PubMed]
- Mulder BA, Defaye P, Boersma LVA. Deep sedation for pulsed field ablation by electrophysiology staff: can and should we do it? Europace 2023;25:euad234. [Crossref] [PubMed]
- Bodys-Pełka A, Kusztal M, Boszko M, et al. Non-Invasive Continuous Measurement of Haemodynamic Parameters-Clinical Utility. J Clin Med 2021;10:4929. [Crossref] [PubMed]
- Patel K, Natale A, Yang R, et al. Is transesophageal echocardiography necessary in patients undergoing ablation of atrial fibrillation on an uninterrupted direct oral anticoagulant regimen? Results from a prospective multicenter registry. Heart Rhythm 2020;17:2093-9. [Crossref] [PubMed]
- Calkins H, Hindricks G, Cappato R, et al. 2017 HRS/EHRA/ECAS/APHRS/SOLAECE expert consensus statement on catheter and surgical ablation of atrial fibrillation. Europace 2018;20:e1-e160. [Crossref] [PubMed]
- Hindricks G, Potpara T, Dagres N, et al. 2020 ESC Guidelines for the diagnosis and management of atrial fibrillation developed in collaboration with the European Association for Cardio-Thoracic Surgery (EACTS): The Task Force for the diagnosis and management of atrial fibrillation of the European Society of Cardiology (ESC) Developed with the special contribution of the European Heart Rhythm Association (EHRA) of the ESC. Eur Heart J 2021;42:373-498. [Crossref] [PubMed]
- Goode JS Jr, Taylor RL, Buffington CW, et al. High-frequency jet ventilation: utility in posterior left atrial catheter ablation. Heart Rhythm 2006;3:13-9. [Crossref] [PubMed]
- Galmén K, Harbut P, Freedman J, et al. High frequency jet ventilation for motion management during ablation procedures, a narrative review. Acta Anaesthesiol Scand 2017;61:1066-74. [Crossref] [PubMed]
- Seiler J, Steven D, Roberts-Thomson KC, et al. The effect of open-irrigated radiofrequency catheter ablation of atrial fibrillation on left atrial pressure and B-type natriuretic peptide. Pacing Clin Electrophysiol 2014;37:616-23. [Crossref] [PubMed]
- Schmidt M, Nölker G, Marschang H, et al. Incidence of oesophageal wall injury post-pulmonary vein antrum isolation for treatment of patients with atrial fibrillation. Europace 2008;10:205-9. [Crossref] [PubMed]
- Di Biase L, Saenz LC, Burkhardt DJ, et al. Esophageal capsule endoscopy after radiofrequency catheter ablation for atrial fibrillation: documented higher risk of luminal esophageal damage with general anesthesia as compared with conscious sedation. Circ Arrhythm Electrophysiol 2009;2:108-12. [Crossref] [PubMed]
- Tschabrunn CM, Attalla S, Salas J, et al. Active esophageal cooling for the prevention of thermal injury during atrial fibrillation ablation: a randomized controlled pilot study. J Interv Card Electrophysiol 2022;63:197-205. [Crossref] [PubMed]
- Tschabrunn CM, Silverstein J, Berzin T, et al. Comparison between single- and multi-sensor oesophageal temperature probes during atrial fibrillation ablation: thermodynamic characteristics. Europace 2015;17:891-7. [Crossref] [PubMed]
- Iwasawa J, Koruth JS, Mittnacht AJ, et al. The impact of mechanical oesophageal deviation on posterior wall pulmonary vein reconnection. Europace 2020;22:232-9. [Crossref] [PubMed]
- Mears JA, Lachman N, Christensen K, et al. The Phrenic Nerve And Atrial Fibrillation Ablation Procedures. J Atr Fibrillation 2009;2:176. [PubMed]
- Calkins H, Kuck KH, Cappato R, et al. 2012 HRS/EHRA/ECAS expert consensus statement on catheter and surgical ablation of atrial fibrillation: recommendations for patient selection, procedural techniques, patient management and follow-up, definitions, endpoints, and research trial design: a report of the Heart Rhythm Society (HRS) Task Force on Catheter and Surgical Ablation of Atrial Fibrillation. Developed in partnership with the European Heart Rhythm Association (EHRA), a registered branch of the European Society of Cardiology (ESC) and the European Cardiac Arrhythmia Society (ECAS); and in collaboration with the American College of Cardiology (ACC), American Heart Association (AHA), the Asia Pacific Heart Rhythm Society (APHRS), and the Society of Thoracic Surgeons (STS). Endorsed by the governing bodies of the American College of Cardiology Foundation, the American Heart Association, the European Cardiac Arrhythmia Society, the European Heart Rhythm Association, the Society of Thoracic Surgeons, the Asia Pacific Heart Rhythm Society, and the Heart Rhythm Society. Heart Rhythm 2012;9:632-696.e21. [Crossref] [PubMed]
- Bessière F, Chevalier P. Pulmonary vein hematoma after atrial fibrillation cryoablation: a new complication. Heart Rhythm 2013;10:1359. [Crossref] [PubMed]
- Hsu LF, Jaïs P, Hocini M, et al. Incidence and prevention of cardiac tamponade complicating ablation for atrial fibrillation. Pacing Clin Electrophysiol 2005;28:S106-9. [Crossref] [PubMed]
- Wyrembak J, Campbell KB, Steinberg BA, et al. Incidence and Predictors of Left Atrial Appendage Thrombus in Patients Treated With Nonvitamin K Oral Anticoagulants Versus Warfarin Before Catheter Ablation for Atrial Fibrillation. Am J Cardiol 2017;119:1017-22. [Crossref] [PubMed]
- Di Biase L, Burkhardt JD, Mohanty P, et al. Periprocedural stroke and management of major bleeding complications in patients undergoing catheter ablation of atrial fibrillation: the impact of periprocedural therapeutic international normalized ratio. Circulation 2010;121:2550-6. [Crossref] [PubMed]
- Canessa R, Lema G, Urzúa J, et al. Anesthesia for elective cardioversion: a comparison of four anesthetic agents. J Cardiothorac Vasc Anesth 1991;5:566-8. [Crossref] [PubMed]
- Wafae BG, da Silva RMF, Veloso HH. Propofol for sedation for direct current cardioversion. Ann Card Anaesth 2019;22:113-21. [Crossref] [PubMed]
- Beaty EH, Fernando RJ, Jacobs ML, et al. Comparison of Bolus Dosing of Methohexital and Propofol in Elective Direct Current Cardioversion. J Am Heart Assoc 2022;11:e026198. [Crossref] [PubMed]
- Kim KM. Remimazolam: pharmacological characteristics and clinical applications in anesthesiology. Anesth Pain Med (Seoul) 2022;17:1-11. [Crossref] [PubMed]
- Sneyd JR, Rigby-Jones AE. Remimazolam for anaesthesia or sedation. Curr Opin Anaesthesiol 2020;33:506-11. [Crossref] [PubMed]
- Karlsson LO, Erixon H, Ebbers T, et al. Post-cardioversion Improvement in LV Function Defined by 4D Flow Patterns and Energetics in Patients With Atrial Fibrillation. Front Physiol 2019;10:659. [Crossref] [PubMed]
- Vladinov G, Fermin L, Longini R, et al. Choosing the anesthetic and sedative drugs for supraventricular tachycardia ablations: A focused review. Pacing Clin Electrophysiol 2018;41:1555-63. [Crossref] [PubMed]
- Brugada J, Katritsis DG, Arbelo E, et al. 2019 ESC Guidelines for the management of patients with supraventricular tachycardiaThe Task Force for the management of patients with supraventricular tachycardia of the European Society of Cardiology (ESC). Eur Heart J 2020;41:655-720. [Crossref] [PubMed]
- Katritsis DG, Zografos T, Katritsis GD, et al. Catheter ablation vs. antiarrhythmic drug therapy in patients with symptomatic atrioventricular nodal re-entrant tachycardia: a randomized, controlled trial. Europace 2017;19:602-6. [PubMed]
- Ho SY. Accessory atrioventricular pathways: getting to the origins. Circulation 2008;117:1502-4. [Crossref] [PubMed]
- Kuck KH, Friday KJ, Kunze KP, et al. Sites of conduction block in accessory atrioventricular pathways. Basis for concealed accessory pathways. Circulation 1990;82:407-17. [Crossref] [PubMed]
- Jackman WM, Wang XZ, Friday KJ, et al. Catheter ablation of accessory atrioventricular pathways (Wolff-Parkinson-White syndrome) by radiofrequency current. N Engl J Med 1991;324:1605-11. [Crossref] [PubMed]
- Katritsis D, Bashir Y, Heald S, et al. Radiofrequency ablation of accessory pathways: implications of accumulated experience and time dedicated to procedures. Eur Heart J 1994;15:339-44. [Crossref] [PubMed]
- Schlüter M, Geiger M, Siebels J, et al. Catheter ablation using radiofrequency current to cure symptomatic patients with tachyarrhythmias related to an accessory atrioventricular pathway. Circulation 1991;84:1644-61. [Crossref] [PubMed]
- Kriebel T, Broistedt C, Kroll M, et al. Efficacy and safety of cryoenergy in the ablation of atrioventricular reentrant tachycardia substrates in children and adolescents. J Cardiovasc Electrophysiol 2005;16:960-6. [Crossref] [PubMed]
- Christoph M, Wunderlich C, Moebius S, et al. Fluoroscopy integrated 3D mapping significantly reduces radiation exposure during ablation for a wide spectrum of cardiac arrhythmias. Europace 2015;17:928-37. [Crossref] [PubMed]
- Mittnacht AJ, Dukkipati S, Mahajan A. Ventricular tachycardia ablation: a comprehensive review for anesthesiologists. Anesth Analg 2015;120:737-48. [Crossref] [PubMed]
- Nof E, Reichlin T, Enriquez AD, et al. Impact of general anesthesia on initiation and stability of VT during catheter ablation. Heart Rhythm 2015;12:2213-20. [Crossref] [PubMed]
- Wutzler A, Mueller A, Loehr L, et al. Minimal and deep sedation during ablation of ventricular tachycardia. Int J Cardiol 2014;172:161-4. [Crossref] [PubMed]
- Ramoul K, Tafer N, Sacher F, et al. Conscious sedation with sufentanil and midazolam for epicardial VT ablation. Journal of Innovations in Cardiac Rhythm Management 2012;3:849-53.
- Mandel JE, Hutchinson MD, Marchlinski FE. Remifentanil-midazolam sedation provides hemodynamic stability and comfort during epicardial ablation of ventricular tachycardia. J Cardiovasc Electrophysiol 2011;22:464-6. [Crossref] [PubMed]
- Kazawa S, Sieira J, Bala G, et al. Impact of anesthetic management on catheter ablation for premature ventricular complexes: insights during the COVID-19 outbreak. J Interv Card Electrophysiol 2023;66:2135-42. [Crossref] [PubMed]
- Cronin EM, Bogun FM, Maury P, et al. 2019 HRS/EHRA/APHRS/LAHRS expert consensus statement on catheter ablation of ventricular arrhythmias. Europace 2019;21:1143-4. [Crossref] [PubMed]
- Turagam MK, Vuddanda V, Atkins D, et al. Hemodynamic Support in Ventricular Tachycardia Ablation: An International VT Ablation Center Collaborative Group Study. JACC Clin Electrophysiol 2017;3:1534-43. [Crossref] [PubMed]
- Marashly Q, Najjar SN, Hahn J, et al. Innovations in ventricular tachycardia ablation. J Interv Card Electrophysiol 2023;66:1499-518. [Crossref] [PubMed]
- Sonny A, Wakefield BJ, Sale S, et al. Transvenous Lead Extraction: A Clinical Commentary for Anesthesiologists. J Cardiothorac Vasc Anesth 2018;32:1101-11. [Crossref] [PubMed]
- Bhatia M, Safavi-Naeini P, Razavi M, et al. Anesthetic Management of Laser Lead Extraction for Cardiovascular Implantable Electronic Devices. Semin Cardiothorac Vasc Anesth 2017;21:302-11. [Crossref] [PubMed]
- Martin A, De Bie B, O'Loughlin J. Anaesthesia for extraction of long-term cardiac device leads. BJA Educ 2022;22:290-4. [Crossref] [PubMed]
- Bongiorni MG, Burri H, Deharo JC, et al. 2018 EHRA expert consensus statement on lead extraction: recommendations on definitions, endpoints, research trial design, and data collection requirements for clinical scientific studies and registries: endorsed by APHRS/HRS/LAHRS. Europace 2018;20:1217. [Crossref] [PubMed]
- Bongiorni MG, Kennergren C, Butter C, et al. The European Lead Extraction ConTRolled (ELECTRa) study: a European Heart Rhythm Association (EHRA) Registry of Transvenous Lead Extraction Outcomes. Eur Heart J 2017;38:2995-3005. [Crossref] [PubMed]
- Azarrafiy R, Tsang DC, Boyle TA, et al. Compliant endovascular balloon reduces the lethality of superior vena cava tears during transvenous lead extractions. Heart Rhythm 2017;14:1400-4. [Crossref] [PubMed]
- Perez AA, Woo FW, Tsang DC, et al. Transvenous Lead Extractions: Current Approaches and Future Trends. Arrhythm Electrophysiol Rev 2018;7:210-7. [Crossref] [PubMed]
- Zsigmond EJ, Saghy L, Benak A, et al. A head-to-head comparison of laser vs. powered mechanical sheaths as first choice and second line extraction tools. Europace 2023;25:591-9. [Crossref] [PubMed]
- Sood N, Martin DT, Lampert R, et al. Incidence and Predictors of Perioperative Complications With Transvenous Lead Extractions: Real-World Experience With National Cardiovascular Data Registry. Circ Arrhythm Electrophysiol 2018;11:e004768. [Crossref] [PubMed]
- Fischer M, Harvey R, Boyle NG, et al. Anesthesia Considerations for Lead Extraction. Card Electrophysiol Clin 2018;10:615-24. [Crossref] [PubMed]
- Maus TM, Shurter J, Nguyen L, et al. Multidisciplinary approach to transvenous lead extraction: a single center's experience. J Cardiothorac Vasc Anesth 2015;29:265-70. [Crossref] [PubMed]
- Husain Z, Safavi-Naeini P, Rasekh A, et al. Anesthetic Management of Patients Undergoing Percutaneous Endocardial and Epicardial Left Atrial Appendage Occlusion. Semin Cardiothorac Vasc Anesth 2017;21:291-301. [Crossref] [PubMed]
- Möbius-Winkler S, Majunke N, Sandri M, et al. Percutaneous left atrial appendage closure: Technical aspects and prevention of periprocedural complications with the watchman device. World J Cardiol 2015;7:65-75. [Crossref] [PubMed]
- Mallouppas M, Vassiliou V. Anticoagulation for Atrial Fibrillation: Is This the End of Warfarin? Not Just Yet. Journal of Angiology 2013;2013:874827. [Crossref]
- Reddy VY, Sievert H, Halperin J, et al. Percutaneous left atrial appendage closure vs warfarin for atrial fibrillation: a randomized clinical trial. JAMA 2014;312:1988-98. [Crossref] [PubMed]
- Holmes DR Jr, Kar S, Price MJ, et al. Prospective randomized evaluation of the Watchman Left Atrial Appendage Closure device in patients with atrial fibrillation versus long-term warfarin therapy: the PREVAIL trial. J Am Coll Cardiol 2014;64:1-12. [Crossref] [PubMed]
- Munir MB, Khan MZ, Darden D, et al. Contemporary procedural trends of Watchman percutaneous left atrial appendage occlusion in the United States. J Cardiovasc Electrophysiol 2021;32:83-92. [Crossref] [PubMed]
- Chan NY, Lau CL, Tsui PT, et al. Experience of left atrial appendage closure performed under conscious sedation. Asian Cardiovasc Thorac Ann 2015;23:394-8. [Crossref] [PubMed]
- Kleinecke C, Allakkis W, Buffle E, et al. Impact of conscious sedation and general anesthesia on periprocedural outcomes in Watchman left atrial appendage closure. Cardiol J 2021;28:519-27. [Crossref] [PubMed]
- Patel A, Venkataraman R, Schurmann P, et al. Left atrial appendage occlusion using intracardiac echocardiography. Heart Rhythm 2021;18:313-7. [Crossref] [PubMed]
- Ramchand J, Harb SC, Miyasaka R, et al. Imaging for percutaneous left atrial appendage closure: A contemporary review. Structural Heart 2019;3:364-82. [Crossref]
- DeLurgio DB, Crossen KJ, Gill J, et al. Hybrid Convergent Procedure for the Treatment of Persistent and Long-Standing Persistent Atrial Fibrillation: Results of CONVERGE Clinical Trial. Circ Arrhythm Electrophysiol 2020;13:e009288. [Crossref] [PubMed]
- Eranki A, Wilson-Smith AR, Williams ML, et al. Hybrid convergent ablation versus endocardial catheter ablation for atrial fibrillation: a systematic review and meta-analysis of randomised control trials and propensity matched studies. J Cardiothorac Surg 2022;17:181. [Crossref] [PubMed]
- McCarthy PM, Cox JL. Author Reply to Commentary: Increasing atrial fibrillation ablation: Insanity is doing the same thing over and over again, but expecting different results. J Thorac Cardiovasc Surg 2024;167:1292. [Crossref] [PubMed]
- Trzcinka A, Lee LS, Madias C, et al. The Convergent Procedure: A Unique Multidisciplinary Hybrid Treatment of Atrial Fibrillation. J Cardiothorac Vasc Anesth 2021;35:631-43. [Crossref] [PubMed]
- Tonks R, Lantz G, Mahlow J, et al. Short and Intermediate Term Outcomes of the Convergent Procedure: Initial Experience in a Tertiary Referral Center. Ann Thorac Cardiovasc Surg 2020;26:13-21. [Crossref] [PubMed]
- DeLurgio DB. The hybrid convergent procedure for persistent and long-standing persistent atrial fibrillation from an electrophysiologist's perspective. J Cardiovasc Electrophysiol 2022;33:1954-60. [Crossref] [PubMed]
- Ahmed A, Pothineni NVK, Charate R, et al. Inappropriate Sinus Tachycardia: Etiology, Pathophysiology, and Management: JACC Review Topic of the Week. J Am Coll Cardiol 2022;79:2450-62. [Crossref] [PubMed]
- Ali M, Haji AQ, Kichloo A, et al. Inappropriate sinus tachycardia: a review. Rev Cardiovasc Med 2021;22:1331-9. [Crossref] [PubMed]
- Sheldon RS, Grubb BP 2nd, Olshansky B, et al. 2015 heart rhythm society expert consensus statement on the diagnosis and treatment of postural tachycardia syndrome, inappropriate sinus tachycardia, and vasovagal syncope. Heart Rhythm 2015;12:e41-63. [Crossref] [PubMed]
- Chiale PA, Garro HA, Schmidberg J, et al. Inappropriate sinus tachycardia may be related to an immunologic disorder involving cardiac beta andrenergic receptors. Heart Rhythm 2006;3:1182-6. [Crossref] [PubMed]
- Morillo CA, Klein GJ, Thakur RK, et al. Mechanism of 'inappropriate' sinus tachycardia. Role of sympathovagal balance. Circulation 1994;90:873-7. [Crossref] [PubMed]
- Mathew ST, Po SS, Thadani U. Inappropriate sinus tachycardia-symptom and heart rate reduction with ivabradine: A pooled analysis of prospective studies. Heart Rhythm 2018;15:240-7. [Crossref] [PubMed]
- Shabtaie SA, Witt CM, Asirvatham SJ. Efficacy of medical and ablation therapy for inappropriate sinus tachycardia: A single-center experience. J Cardiovasc Electrophysiol 2021;32:1053-61. [Crossref] [PubMed]
- Marrouche NF, Beheiry S, Tomassoni G, et al. Three-dimensional nonfluoroscopic mapping and ablation of inappropriate sinus tachycardia. Procedural strategies and long-term outcome. J Am Coll Cardiol 2002;39:1046-54. [Crossref] [PubMed]
- Lee RJ, Kalman JM, Fitzpatrick AP, et al. Radiofrequency catheter modification of the sinus node for "inappropriate" sinus tachycardia. Circulation 1995;92:2919-28. [Crossref] [PubMed]
- Rodríguez-Mañero M, Kreidieh B, Al Rifai M, et al. Ablation of Inappropriate Sinus Tachycardia: A Systematic Review of the Literature. JACC Clin Electrophysiol 2017;3:253-65. [Crossref] [PubMed]
- Olshansky B, Sullivan RM. Inappropriate sinus tachycardia. J Am Coll Cardiol 2013;61:793-801. [Crossref] [PubMed]
Cite this article as: Hicks MH, Marchant BE, Rajkumar KP, Coleman SR, Fernando RJ, Morgan TR, Kozak PM, Royster RL. Anesthesia for electrophysiology procedures. AME Med J 2025;10:24.